
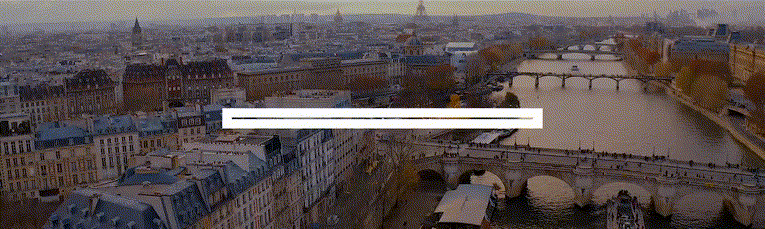
Is Time Travel Possible?
We all travel in time! We travel one year in time between birthdays, for example. And we are all traveling in time at approximately the same speed: 1 second per second.
We typically experience time at one second per second. Credit: NASA/JPL-Caltech
NASA's space telescopes also give us a way to look back in time. Telescopes help us see stars and galaxies that are very far away . It takes a long time for the light from faraway galaxies to reach us. So, when we look into the sky with a telescope, we are seeing what those stars and galaxies looked like a very long time ago.
However, when we think of the phrase "time travel," we are usually thinking of traveling faster than 1 second per second. That kind of time travel sounds like something you'd only see in movies or science fiction books. Could it be real? Science says yes!
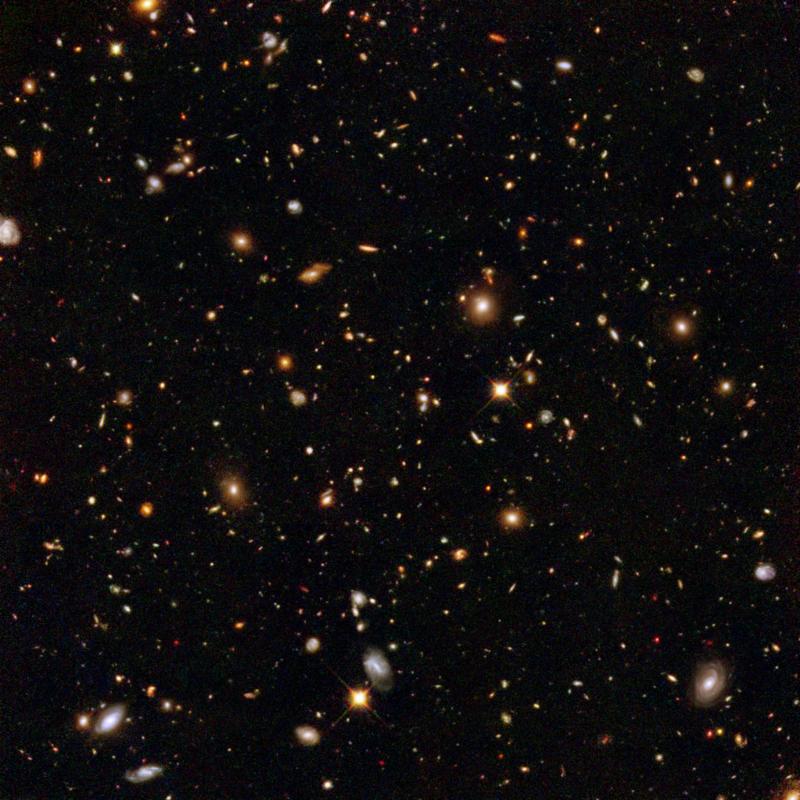
This image from the Hubble Space Telescope shows galaxies that are very far away as they existed a very long time ago. Credit: NASA, ESA and R. Thompson (Univ. Arizona)
How do we know that time travel is possible?
More than 100 years ago, a famous scientist named Albert Einstein came up with an idea about how time works. He called it relativity. This theory says that time and space are linked together. Einstein also said our universe has a speed limit: nothing can travel faster than the speed of light (186,000 miles per second).
Einstein's theory of relativity says that space and time are linked together. Credit: NASA/JPL-Caltech
What does this mean for time travel? Well, according to this theory, the faster you travel, the slower you experience time. Scientists have done some experiments to show that this is true.
For example, there was an experiment that used two clocks set to the exact same time. One clock stayed on Earth, while the other flew in an airplane (going in the same direction Earth rotates).
After the airplane flew around the world, scientists compared the two clocks. The clock on the fast-moving airplane was slightly behind the clock on the ground. So, the clock on the airplane was traveling slightly slower in time than 1 second per second.
Credit: NASA/JPL-Caltech
Can we use time travel in everyday life?
We can't use a time machine to travel hundreds of years into the past or future. That kind of time travel only happens in books and movies. But the math of time travel does affect the things we use every day.
For example, we use GPS satellites to help us figure out how to get to new places. (Check out our video about how GPS satellites work .) NASA scientists also use a high-accuracy version of GPS to keep track of where satellites are in space. But did you know that GPS relies on time-travel calculations to help you get around town?
GPS satellites orbit around Earth very quickly at about 8,700 miles (14,000 kilometers) per hour. This slows down GPS satellite clocks by a small fraction of a second (similar to the airplane example above).
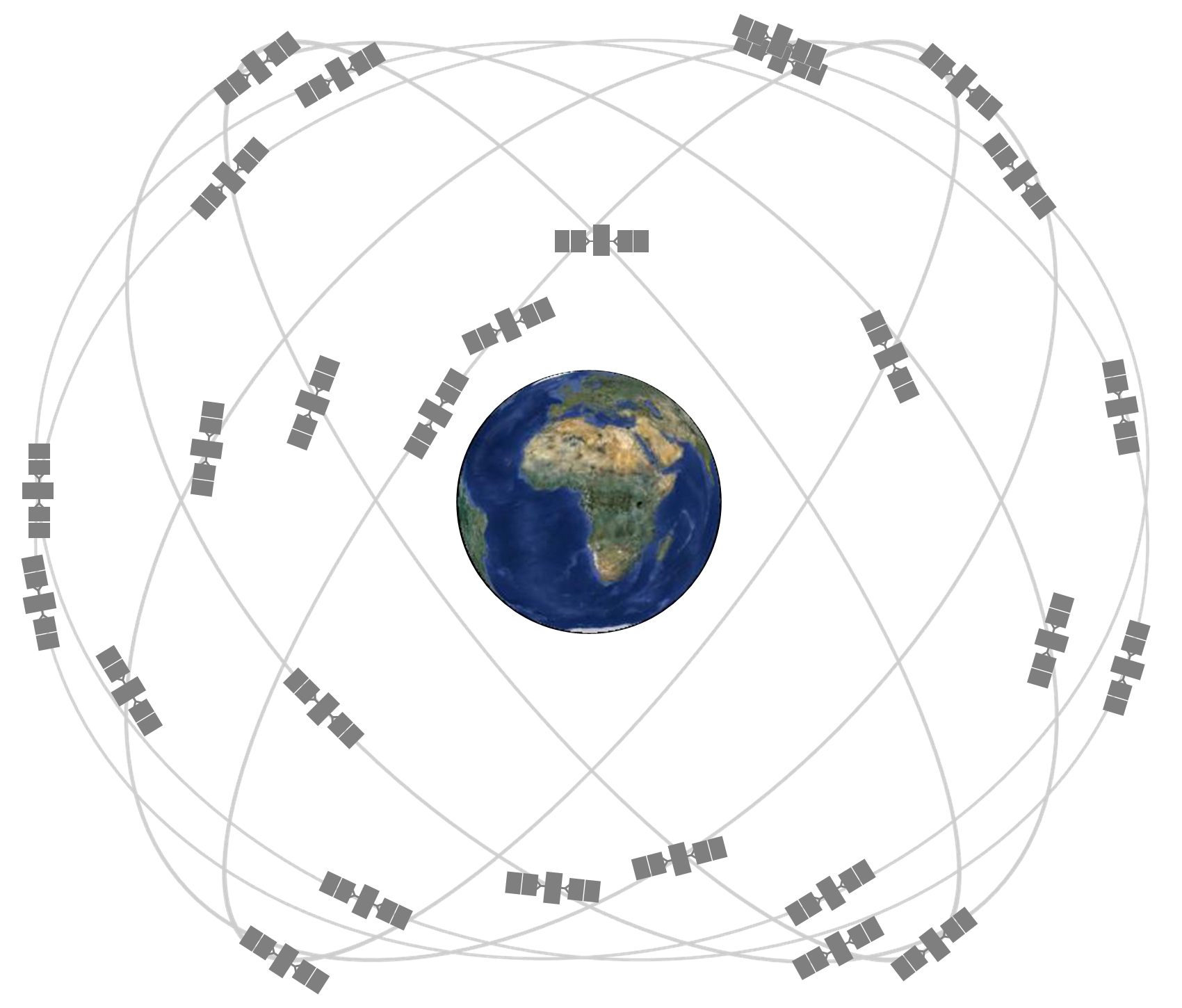
GPS satellites orbit around Earth at about 8,700 miles (14,000 kilometers) per hour. Credit: GPS.gov
However, the satellites are also orbiting Earth about 12,550 miles (20,200 km) above the surface. This actually speeds up GPS satellite clocks by a slighter larger fraction of a second.
Here's how: Einstein's theory also says that gravity curves space and time, causing the passage of time to slow down. High up where the satellites orbit, Earth's gravity is much weaker. This causes the clocks on GPS satellites to run faster than clocks on the ground.
The combined result is that the clocks on GPS satellites experience time at a rate slightly faster than 1 second per second. Luckily, scientists can use math to correct these differences in time.
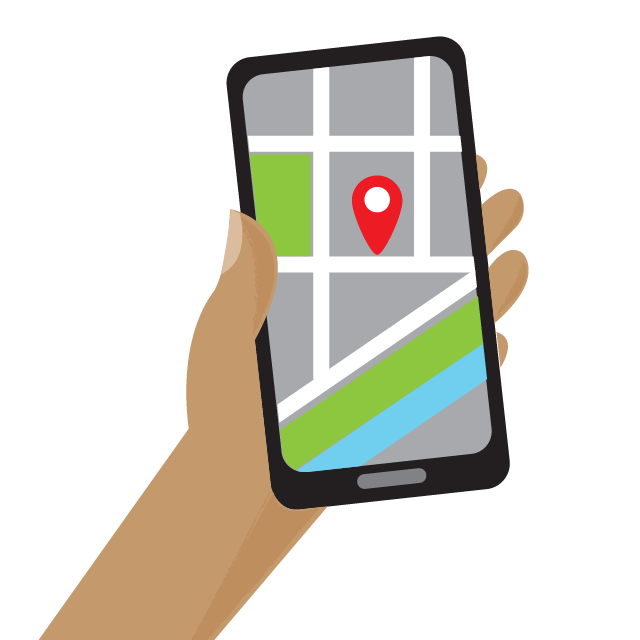
If scientists didn't correct the GPS clocks, there would be big problems. GPS satellites wouldn't be able to correctly calculate their position or yours. The errors would add up to a few miles each day, which is a big deal. GPS maps might think your home is nowhere near where it actually is!
In Summary:
Yes, time travel is indeed a real thing. But it's not quite what you've probably seen in the movies. Under certain conditions, it is possible to experience time passing at a different rate than 1 second per second. And there are important reasons why we need to understand this real-world form of time travel.
If you liked this, you may like:


We Already Know How to Build a Time Machine, Scientists Say
It’s just a matter of time before we build one that can take us into the far future.
“When Mr. Padalka came back from his adventures, he found the Earth to be 1/44th of a second to the future of where he expected it to be,” explains J. Richard Gott, Princeton physicist and author of the 2001 book Time Travel in Einstein’s Universe . “He literally traveled ... into the future.”
While being a fraction of a second younger than if he had stayed on Earth isn’t mind-bending stuff, it nonetheless gave Padalka the distinction of the “current time traveler record,” according to Gott.
Although not exactly a plutonium-charged DeLorean , time travel is anything but fiction. Real astrophysicists like Gott are pretty sure they know how to build a time machine, and intense speed—much, much faster than Padalka’s orbital jaunt—is the key ingredient.
A Brief Crash Course on Time Travel
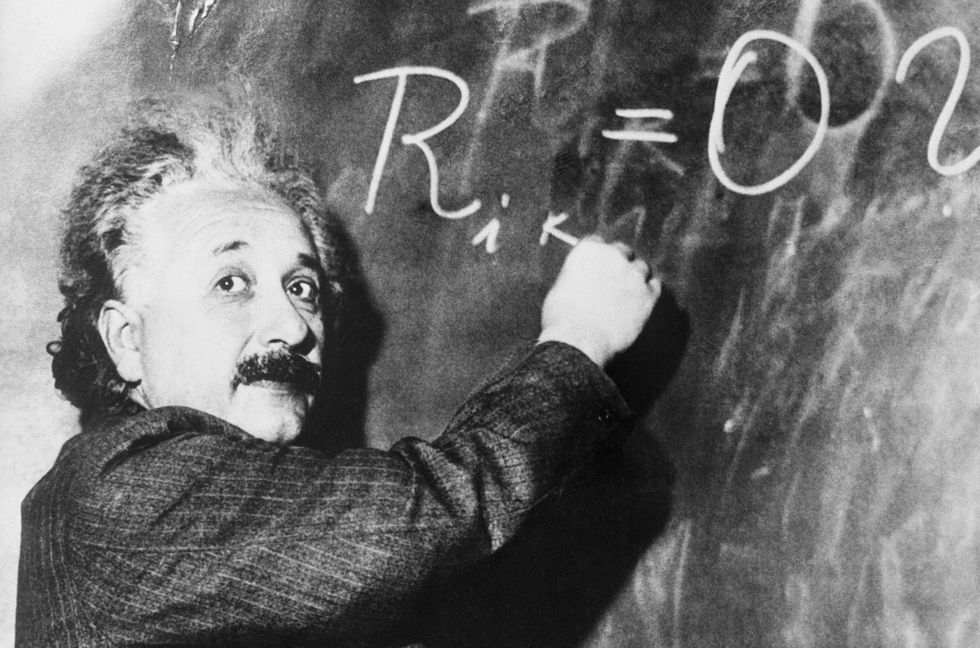
Until the 20th century, time was believed to be completely immutable, and time travel a scientific impossibility. In the 1680s, Sir Isaac Newton’s thought time progressed at a consistent pace throughout the universe, regardless of outside forces or location. And for two centuries, the scientific world subscribed to Newton’s theory.
That is, until 26-year-old Albert Einstein came along.
In 1905, Einstein revealed his ideas on special relativity , using this framework for his theory of general relativity a decade later. Einstein’s universe-defining calculations introduced, well , lots of things, but also some concepts related to time. The most important being that time is elastic and dependent on speed, slowing down or speeding up depending on how fast an object—or person—is moving.
.css-2l0eat{font-family:UnitedSans,UnitedSans-roboto,UnitedSans-local,Helvetica,Arial,Sans-serif;font-size:1.625rem;line-height:1.2;margin:0rem;padding:0.9rem 1rem 1rem;}@media(max-width: 48rem){.css-2l0eat{font-size:1.75rem;line-height:1;}}@media(min-width: 48rem){.css-2l0eat{font-size:1.875rem;line-height:1;}}@media(min-width: 64rem){.css-2l0eat{font-size:2.25rem;line-height:1;}}.css-2l0eat b,.css-2l0eat strong{font-family:inherit;font-weight:bold;}.css-2l0eat em,.css-2l0eat i{font-style:italic;font-family:inherit;} “Without Einstein’s general theory of relatively, our GPS system wouldn’t be working.”
In 1971, four cesium-beam atomic clocks flew around the world and were then compared to ground-based clocks. The resulting minuscule time difference proved that Einstein was onto something. There’s also another technology, tucked inside your smartphone, that also validates Einstein’s theory.
“Without Einstein’s general theory of relatively, our GPS system wouldn’t be working,” says Ron Mallet, an astrophysicist and author of the book Time Traveler: A Scientist’s Personal Mission to Make Time Travel a Reality. “That’s also proof that Einstein’s [theories are] correct.”
But apart from this mutable version of time, Einstein also calculated the speed of light . At 300,000,000 meters (or 186,282 miles) per second, Einstein describes the figure as the “ultimate speed limit” and a universal constant no matter if one is sitting on a bench or traveling in a rocket ship.
The last bit of Einstein’s time-bending ideas suggest that gravity also slows time, meaning time runs faster where gravity is weaker, like the vast emptiness among massive celestial bodies like the sun, Jupiter, and Earth .
Fast forward a century later, and all of these theories—highly summarized, of course—now form the building blocks of astrophysics , and buried among all this expert-level math, Einstein also proved that time travel was possible.
The Subatomic Time Machine
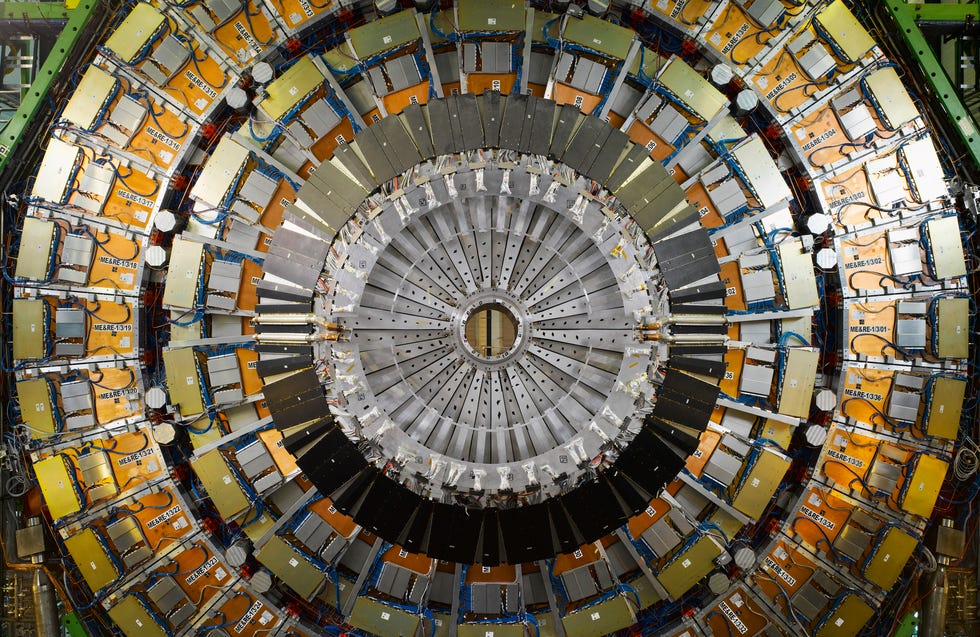
In fact, not only is time travel possible, it’s already happened—it just doesn’t look like your typical sci-fi film .
Returning to our time-traveling cosmonaut Padalka, his 1/44th-second jump into the future is so minuscule because he was only traveling at 17,000 miles per hour. That isn’t very fast, at least in comparison to the speed of light. But what would happen if we created something that could go much faster than geostationary orbit? We’re not talking a commercial jetliner (550 to 600 miles an hour) or a 21st-century rocket to the ISS (25,000 miles per hour), but something that could approach 186,282 miles per second.
“On a subatomic level, it’s been done,” says Mallett. “An example is ... the Large Hadron Collider . It routinely sends subatomic particles into the future.”
The particle accelerator has the ability to propel protons at 99.999999 percent the speed of light, a speed at which their relative time is moving about 6,900 times slower compared to their stationary human observers.
“The Large Hadron Collider ... routinely sends subatomic particles into the future.”
So, yes, we’ve been sending atoms into the future and we’ve been doing it for the last decade, but humans are another matter.
Gott says given that we propel particles nearly the speed of light on a regular basis, conceptually, it’s rather simple for humans to time travel into the future. “If you want to visit Earth in the year 3000,” Gott says, “all you have to do is to get on a spaceship and go 99.995 percent the speed of light.”
Let’s say a human is put on such a ship and sent to a planet that’s a little less than 500 light years away (for example, Kepler 186f ), meaning if they traveled at 99.995 percent of the speed of light, it would take them about 500 years to get there, since they are going at nearly the speed of light.
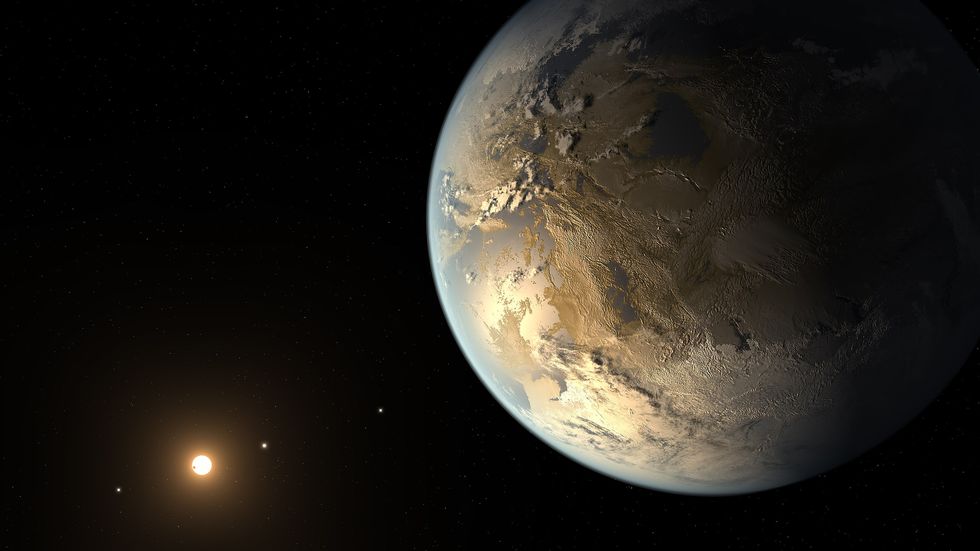
After a quick snack and a bathroom break, they would then turn around and head back to Earth, which would take another 500 years. So in total, it would take about 1,000 years for them to arrive safely back home. And, on Earth, it would be the year 3022.
However, since they were moving so fast, the resulting time dilation wouldn’t seem like 1,000 years for them, since their internal clock has slowed. “[Their] clock will be ticking at 1/100th of the rate of the clocks on Earth. [They] are only going to age about 10 years,” says Gott. While a millennium would pass for us, for them it would be a decade.
“If we [on Earth] were watching through the window, they would be eating breakfast veeeerrry slooooowly,” says Gott, “But to [them], everything would be normal.”
But there is a massive gulf between what is theoretical and what is real. So how do we overcome the immense technological challenges of how to build a time machine?
The Not-So-Distant Future of Human Time Travel
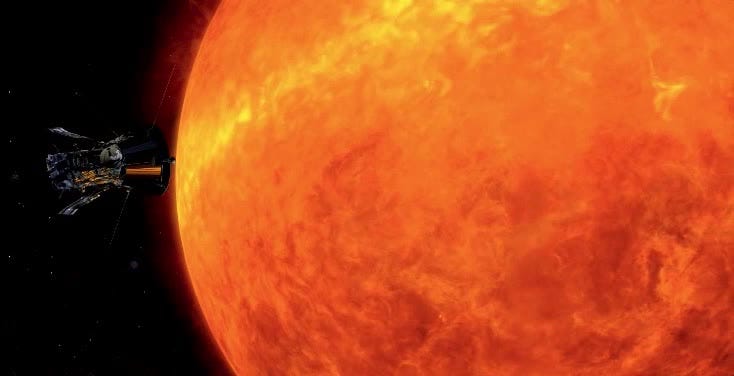
Building a time-traveling spaceship may be the best place to start, but the engineering obstacles, at least for now, are enormous. For one, we are not even close to having a spaceship that can travel at the speed of light. The fastest spacecraft ever created is the Parker Solar Probe , which launched in 2018 with the mission to study the sun’s outer corona. It travels only .00067 percent the speed of light .
There’s also the enormous amount of energy that would be needed to propel a ship to go that fast. Gott suggests that highly efficient antimatter fuel could be the key; other world agencies and scientists also think such a fuel could be a potentially invaluable piece to interstellar travel .
But ensuring the safety of human cargo on such a futuristic mission would also be tricky. First of all, the ship would need to carry enough supplies—like food, water, and medicine— and be self-sufficient for the entire journey.
✅ Get the Facts: Time Travel
- The Infamous ‘Grandfather Paradox’ Doesn’t Make Time Travel Impossible After All
- Time Travel Movies Rely on the ‘Bootstrap Paradox.’ It Could Explain Real-Life Destiny
- A Student Just Proved Paradox-Free Time Travel Is Possible
- The 35 Best Time Travel Movies
Then there’s the whole acceleration thing. To make sure our hypothetical traveler wouldn’t be obliterated by overwhelming g forces, the ship would need to gradually and steadily accelerate. While steady 1g acceleration (like what we feel on Earth) for a long period of time would eventually get the ship to approach near speed of light, it would add to the length of the trip and minimize how far in the future one could go.
Using our 500-light-year planet example, Gott predicts that the steady acceleration of 1g up to near light speed would increase the aging of the time traveler to 24 years, “but you would still get to visit Earth in the year 3000,” says Gott.
To create a vehicle with these specifications would require a lot of time, resources, and money. But the same can be said for other massively ambitious experiments, like detecting gravitational waves and building the Large Hadron Collider. A time machine could be the world’s next scientific megaproject .
The Trouble of Going in Reverse
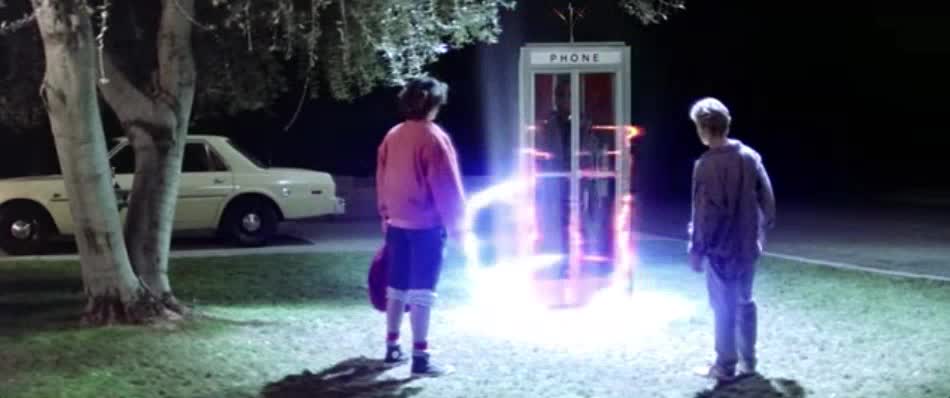
But there is one big caveat to this theoretical portrait of real-world time travel— this machine doesn’t go in reverse . While Bill and Ted travel to the past to pick up Socrates with relative ease, in reality, scientists and researchers need to find a way to circumvent the rules of physics in order to travel back in time.
Wormholes , black holes, cosmic strings , and circulating light beams have all been suggested as potential solutions for time-traveling to the past. The main challenge that astrophysicists are grappling with is figuring out is how to beat a light beam to a point in spacetime and back.
“The technology isn’t far off ... we could do it in the next twenty years.”
Since the speed of light is the absolute maximum, physicists are concentrating on finding phenomena like wormholes, which could provide tunnel-like shortcuts that jump across curved spacetime and, in theory, beat a light beam to a particular point in spacetime.
While wormholes do work within the confines of Einstein’s theories of relativity, they have yet to be observed in space, and scientists have no concrete evidence that these galactic shortcuts would even work .
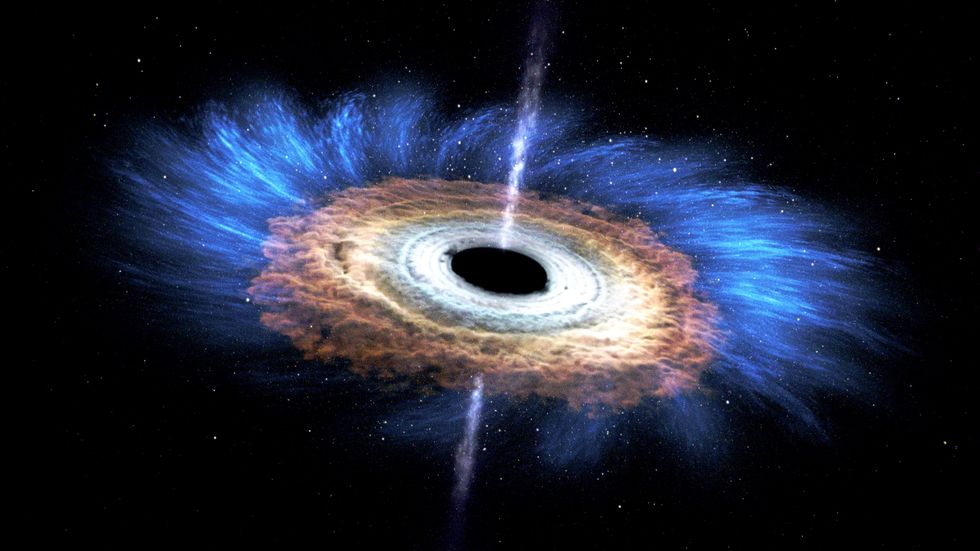
So while time traveling to the past may be the more exciting concept, scientists are much more likely to fling someone into the unknown future rather than the well-trodden past. But despite overwhelming odds—fiscal and scientific—Mallet believes the future of a time-traveling society is possible.
“What happened with going to the moon ... we wanted to go there, Kennedy asked for it, and there was proper funding so we got there within a decade,” Mallet says. “The technology isn’t far off. If the government and taxpayers wanted to pay for it, we could do it in the next 20 years.”
For now, wannabe time travelers will still have to look to science fiction for a time travel fix, with some movies being much more accurate than others.
“A good movie ... was the original Planet of the Apes ,” says Mallett. “The astronauts thought they had landed on another planet that was ruled by apes, but what they found out ... was that they had traveled so fast, that they had arrived into Earth’s future . That movie accurately depicts Einstein’s special theory of relativity.”
Oh ... spoilers.
Matt is a history, science, and travel writer who is always searching for the mysterious and hidden. He's written for Smithsonian Magazine, Washingtonian, Atlas Obscura, and Arlington Magazine. He calls Washington D.C. home and probably tells way too many cat jokes.
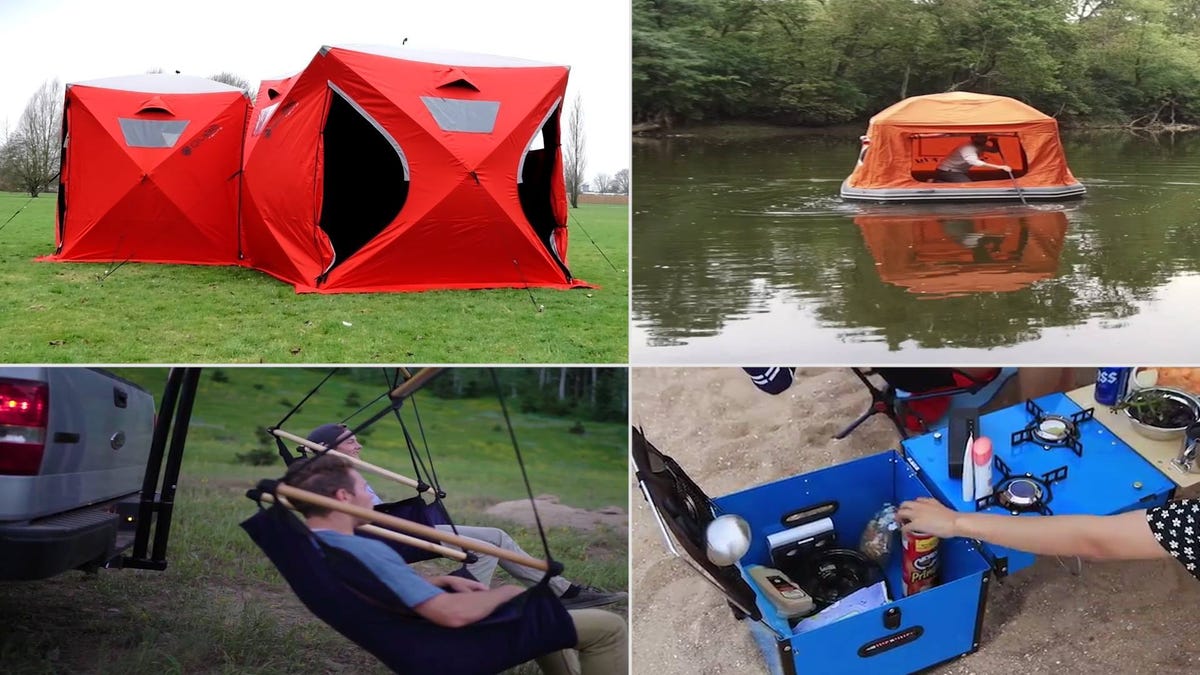
.css-cuqpxl:before{padding-right:0.3125rem;content:'//';display:inline;} The Story of Science Fiction .css-xtujxj:before{padding-left:0.3125rem;content:'//';display:inline;}
The 2020 Sci-Fi Film Guide
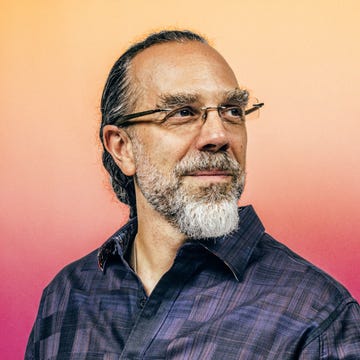
Why You Shouldn't Fear AI
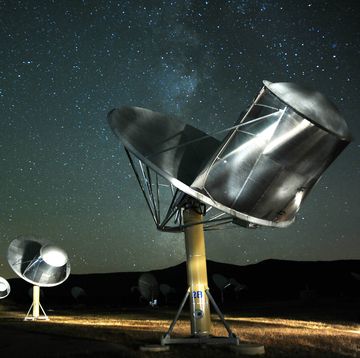
A Look at the Epic 50-Year Search for E.T.
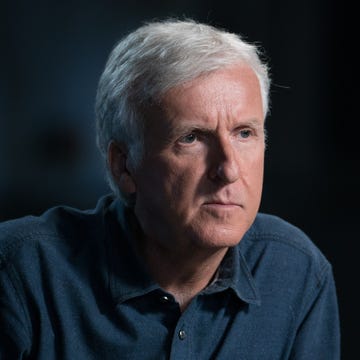
James Cameron Bringing the History of Sci-Fi To TV
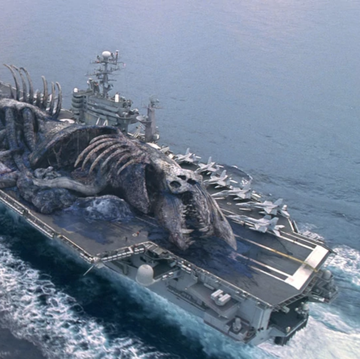
How the Pentagon Could Destroy All Monsters
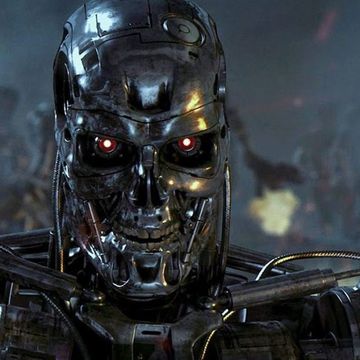
Google Is Working on Plans to Prevent Skynet
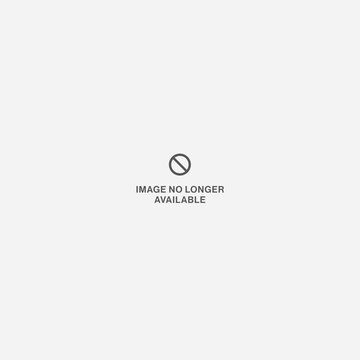
The Impossible Anatomy of Godzilla
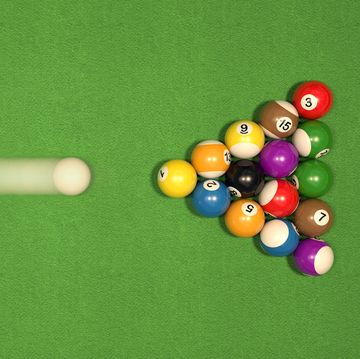
Billiards With Memory Creates Intricate Patterns
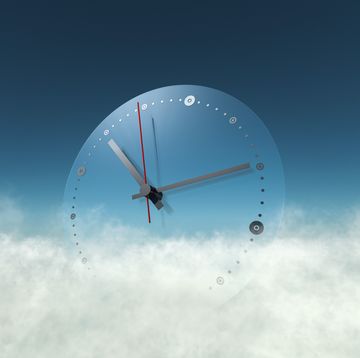
A Wonder Clock Has Rocked the Scientific World
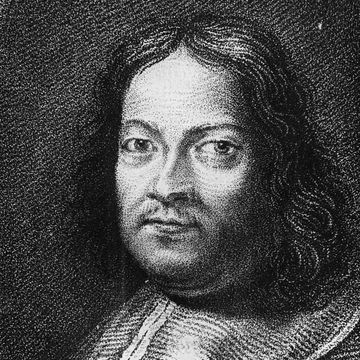
Machines About to Tackle Fermat’s Last Theorem
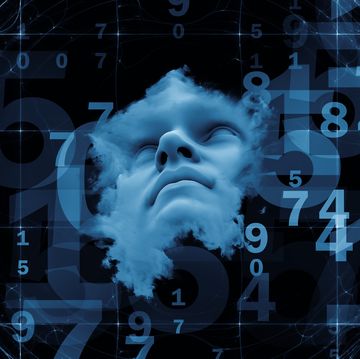
Can AI Help Solve Math’s Thorniest Mysteries?
September 2, 2014
Time Travel Simulation Resolves “Grandfather Paradox”
What would happen to you if you went back in time and killed your grandfather? A model using photons reveals that quantum mechanics can solve the quandary—and even foil quantum cryptography
By Lee Billings
On June 28, 2009, the world-famous physicist Stephen Hawking threw a party at the University of Cambridge, complete with balloons, hors d'oeuvres and iced champagne. Everyone was invited but no one showed up. Hawking had expected as much, because he only sent out invitations after his party had concluded. It was, he said, "a welcome reception for future time travelers," a tongue-in-cheek experiment to reinforce his 1992 conjecture that travel into the past is effectively impossible.
But Hawking may be on the wrong side of history. Recent experiments offer tentative support for time travel's feasibility—at least from a mathematical perspective. The study cuts to the core of our understanding of the universe, and the resolution of the possibility of time travel, far from being a topic worthy only of science fiction, would have profound implications for fundamental physics as well as for practical applications such as quantum cryptography and computing.
Closed timelike curves The source of time travel speculation lies in the fact that our best physical theories seem to contain no prohibitions on traveling backward through time. The feat should be possible based on Einstein's theory of general relativity, which describes gravity as the warping of spacetime by energy and matter. An extremely powerful gravitational field, such as that produced by a spinning black hole, could in principle profoundly warp the fabric of existence so that spacetime bends back on itself. This would create a "closed timelike curve," or CTC, a loop that could be traversed to travel back in time.
On supporting science journalism
If you're enjoying this article, consider supporting our award-winning journalism by subscribing . By purchasing a subscription you are helping to ensure the future of impactful stories about the discoveries and ideas shaping our world today.
Hawking and many other physicists find CTCs abhorrent, because any macroscopic object traveling through one would inevitably create paradoxes where cause and effect break down. In a model proposed by the theorist David Deutsch in 1991, however, the paradoxes created by CTCs could be avoided at the quantum scale because of the behavior of fundamental particles, which follow only the fuzzy rules of probability rather than strict determinism. "It's intriguing that you've got general relativity predicting these paradoxes, but then you consider them in quantum mechanical terms and the paradoxes go away," says University of Queensland physicist Tim Ralph. "It makes you wonder whether this is important in terms of formulating a theory that unifies general relativity with quantum mechanics."
Experimenting with a curve Recently Ralph and his PhD student Martin Ringbauer led a team that experimentally simulated Deutsch's model of CTCs for the very first time, testing and confirming many aspects of the two-decades-old theory. Their findings are published in Nature Communications. Much of their simulation revolved around investigating how Deutsch's model deals with the “grandfather paradox,” a hypothetical scenario in which someone uses a CTC to travel back through time to murder her own grandfather, thus preventing her own later birth. ( Scientific American is part of Nature Publishing Group.)
Deutsch's quantum solution to the grandfather paradox works something like this:
Instead of a human being traversing a CTC to kill her ancestor, imagine that a fundamental particle goes back in time to flip a switch on the particle-generating machine that created it. If the particle flips the switch, the machine emits a particle— the particle—back into the CTC; if the switch isn't flipped, the machine emits nothing. In this scenario there is no a priori deterministic certainty to the particle's emission, only a distribution of probabilities. Deutsch's insight was to postulate self-consistency in the quantum realm, to insist that any particle entering one end of a CTC must emerge at the other end with identical properties. Therefore, a particle emitted by the machine with a probability of one half would enter the CTC and come out the other end to flip the switch with a probability of one half, imbuing itself at birth with a probability of one half of going back to flip the switch. If the particle were a person, she would be born with a one-half probability of killing her grandfather, giving her grandfather a one-half probability of escaping death at her hands—good enough in probabilistic terms to close the causative loop and escape the paradox. Strange though it may be, this solution is in keeping with the known laws of quantum mechanics.
In their new simulation Ralph, Ringbauer and their colleagues studied Deutsch's model using interactions between pairs of polarized photons within a quantum system that they argue is mathematically equivalent to a single photon traversing a CTC. "We encode their polarization so that the second one acts as kind of a past incarnation of the first,” Ringbauer says. So instead of sending a person through a time loop, they created a stunt double of the person and ran him through a time-loop simulator to see if the doppelganger emerging from a CTC exactly resembled the original person as he was in that moment in the past.
By measuring the polarization states of the second photon after its interaction with the first, across multiple trials the team successfully demonstrated Deutsch's self-consistency in action. "The state we got at our output, the second photon at the simulated exit of the CTC, was the same as that of our input, the first encoded photon at the CTC entrance," Ralph says. "Of course, we're not really sending anything back in time but [the simulation] allows us to study weird evolutions normally not allowed in quantum mechanics."
Those "weird evolutions" enabled by a CTC, Ringbauer notes, would have remarkable practical applications, such as breaking quantum-based cryptography through the cloning of the quantum states of fundamental particles. "If you can clone quantum states,” he says, “you can violate the Heisenberg uncertainty principle,” which comes in handy in quantum cryptography because the principle forbids simultaneously accurate measurements of certain kinds of paired variables, such as position and momentum. "But if you clone that system, you can measure one quantity in the first and the other quantity in the second, allowing you to decrypt an encoded message."
"In the presence of CTCs, quantum mechanics allows one to perform very powerful information-processing tasks, much more than we believe classical or even normal quantum computers could do," says Todd Brun, a physicist at the University of Southern California who was not involved with the team's experiment. "If the Deutsch model is correct, then this experiment faithfully simulates what could be done with an actual CTC. But this experiment cannot test the Deutsch model itself; that could only be done with access to an actual CTC."
Alternative reasoning Deutsch's model isn’t the only one around, however. In 2009 Seth Lloyd, a theorist at Massachusetts Institute of Technology, proposed an alternative , less radical model of CTCs that resolves the grandfather paradox using quantum teleportation and a technique called post-selection, rather than Deutsch's quantum self-consistency. With Canadian collaborators, Lloyd went on to perform successful laboratory simulations of his model in 2011. "Deutsch's theory has a weird effect of destroying correlations," Lloyd says. "That is, a time traveler who emerges from a Deutschian CTC enters a universe that has nothing to do with the one she exited in the future. By contrast, post-selected CTCs preserve correlations, so that the time traveler returns to the same universe that she remembers in the past."
This property of Lloyd's model would make CTCs much less powerful for information processing, although still far superior to what computers could achieve in typical regions of spacetime. "The classes of problems our CTCs could help solve are roughly equivalent to finding needles in haystacks," Lloyd says. "But a computer in a Deutschian CTC could solve why haystacks exist in the first place.”
Lloyd, though, readily admits the speculative nature of CTCs. “I have no idea which model is really right. Probably both of them are wrong,” he says. Of course, he adds, the other possibility is that Hawking is correct, “that CTCs simply don't and cannot exist." Time-travel party planners should save the champagne for themselves—their hoped-for future guests seem unlikely to arrive.
The Quantum Physics of Time Travel (All-Access Subscribers Only) By David Deutsch and Michael Lockwood
Can Quantum Bayesianism Fix the Paradoxes of Quantum Mechanics?
Astrophysicist J. Richard Gott on Time Travel
Is time travel possible? Why one scientist says we 'cannot ignore the possibility.'

A common theme in science-fiction media , time travel is captivating. It’s defined by the late philosopher David Lewis in his essay “The Paradoxes of Time Travel” as “[involving] a discrepancy between time and space time. Any traveler departs and then arrives at his destination; the time elapsed from departure to arrival … is the duration of the journey.”
Time travel is usually understood by most as going back to a bygone era or jumping forward to a point far in the future . But how much of the idea is based in reality? Is it possible to travel through time?
Is time travel possible?
According to NASA, time travel is possible , just not in the way you might expect. Albert Einstein’s theory of relativity says time and motion are relative to each other, and nothing can go faster than the speed of light , which is 186,000 miles per second. Time travel happens through what’s called “time dilation.”
Time dilation , according to Live Science, is how one’s perception of time is different to another's, depending on their motion or where they are. Hence, time being relative.
Learn more: Best travel insurance
Dr. Ana Alonso-Serrano, a postdoctoral researcher at the Max Planck Institute for Gravitational Physics in Germany, explained the possibility of time travel and how researchers test theories.
Space and time are not absolute values, Alonso-Serrano said. And what makes this all more complex is that you are able to carve space-time .
“In the moment that you carve the space-time, you can play with that curvature to make the time come in a circle and make a time machine,” Alonso-Serrano told USA TODAY.
She explained how, theoretically, time travel is possible. The mathematics behind creating curvature of space-time are solid, but trying to re-create the strict physical conditions needed to prove these theories can be challenging.
“The tricky point of that is if you can find a physical, realistic, way to do it,” she said.
Alonso-Serrano said wormholes and warp drives are tools that are used to create this curvature. The matter needed to achieve curving space-time via a wormhole is exotic matter , which hasn’t been done successfully. Researchers don’t even know if this type of matter exists, she said.
“It's something that we work on because it's theoretically possible, and because it's a very nice way to test our theory, to look for possible paradoxes,” Alonso-Serrano added.
“I could not say that nothing is possible, but I cannot ignore the possibility,” she said.
She also mentioned the anecdote of Stephen Hawking’s Champagne party for time travelers . Hawking had a GPS-specific location for the party. He didn’t send out invites until the party had already happened, so only people who could travel to the past would be able to attend. No one showed up, and Hawking referred to this event as "experimental evidence" that time travel wasn't possible.
What did Albert Einstein invent?: Discoveries that changed the world
Just Curious for more? We've got you covered
USA TODAY is exploring the questions you and others ask every day. From "How to watch the Marvel movies in order" to "Why is Pluto not a planet?" to "What to do if your dog eats weed?" – we're striving to find answers to the most common questions you ask every day. Head to our Just Curious section to see what else we can answer for you.
A beginner's guide to time travel
Learn exactly how Einstein's theory of relativity works, and discover how there's nothing in science that says time travel is impossible.
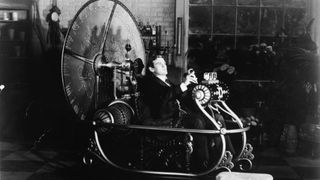
Everyone can travel in time . You do it whether you want to or not, at a steady rate of one second per second. You may think there's no similarity to traveling in one of the three spatial dimensions at, say, one foot per second. But according to Einstein 's theory of relativity , we live in a four-dimensional continuum — space-time — in which space and time are interchangeable.
Einstein found that the faster you move through space, the slower you move through time — you age more slowly, in other words. One of the key ideas in relativity is that nothing can travel faster than the speed of light — about 186,000 miles per second (300,000 kilometers per second), or one light-year per year). But you can get very close to it. If a spaceship were to fly at 99% of the speed of light, you'd see it travel a light-year of distance in just over a year of time.
That's obvious enough, but now comes the weird part. For astronauts onboard that spaceship, the journey would take a mere seven weeks. It's a consequence of relativity called time dilation , and in effect, it means the astronauts have jumped about 10 months into the future.
Traveling at high speed isn't the only way to produce time dilation. Einstein showed that gravitational fields produce a similar effect — even the relatively weak field here on the surface of Earth . We don't notice it, because we spend all our lives here, but more than 12,400 miles (20,000 kilometers) higher up gravity is measurably weaker— and time passes more quickly, by about 45 microseconds per day. That's more significant than you might think, because it's the altitude at which GPS satellites orbit Earth, and their clocks need to be precisely synchronized with ground-based ones for the system to work properly.
The satellites have to compensate for time dilation effects due both to their higher altitude and their faster speed. So whenever you use the GPS feature on your smartphone or your car's satnav, there's a tiny element of time travel involved. You and the satellites are traveling into the future at very slightly different rates.
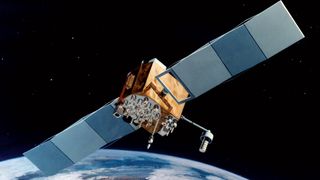
But for more dramatic effects, we need to look at much stronger gravitational fields, such as those around black holes , which can distort space-time so much that it folds back on itself. The result is a so-called wormhole, a concept that's familiar from sci-fi movies, but actually originates in Einstein's theory of relativity. In effect, a wormhole is a shortcut from one point in space-time to another. You enter one black hole, and emerge from another one somewhere else. Unfortunately, it's not as practical a means of transport as Hollywood makes it look. That's because the black hole's gravity would tear you to pieces as you approached it, but it really is possible in theory. And because we're talking about space-time, not just space, the wormhole's exit could be at an earlier time than its entrance; that means you would end up in the past rather than the future.
Trajectories in space-time that loop back into the past are given the technical name "closed timelike curves." If you search through serious academic journals, you'll find plenty of references to them — far more than you'll find to "time travel." But in effect, that's exactly what closed timelike curves are all about — time travel
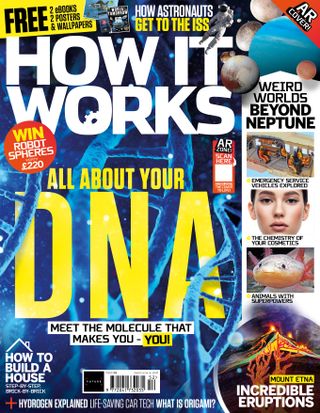
This article is brought to you by How It Works .
How It Works is the action-packed magazine that's bursting with exciting information about the latest advances in science and technology, featuring everything you need to know about how the world around you — and the universe — works.
There's another way to produce a closed timelike curve that doesn't involve anything quite so exotic as a black hole or wormhole: You just need a simple rotating cylinder made of super-dense material. This so-called Tipler cylinder is the closest that real-world physics can get to an actual, genuine time machine. But it will likely never be built in the real world, so like a wormhole, it's more of an academic curiosity than a viable engineering design.
Yet as far-fetched as these things are in practical terms, there's no fundamental scientific reason — that we currently know of — that says they are impossible. That's a thought-provoking situation, because as the physicist Michio Kaku is fond of saying, "Everything not forbidden is compulsory" (borrowed from T.H. White's novel, "The Once And Future King"). He doesn't mean time travel has to happen everywhere all the time, but Kaku is suggesting that the universe is so vast it ought to happen somewhere at least occasionally. Maybe some super-advanced civilization in another galaxy knows how to build a working time machine, or perhaps closed timelike curves can even occur naturally under certain rare conditions.
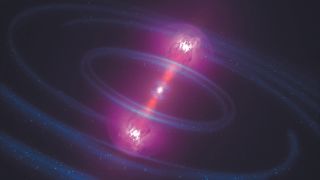
This raises problems of a different kind — not in science or engineering, but in basic logic. If time travel is allowed by the laws of physics, then it's possible to envision a whole range of paradoxical scenarios . Some of these appear so illogical that it's difficult to imagine that they could ever occur. But if they can't, what's stopping them?
Thoughts like these prompted Stephen Hawking , who was always skeptical about the idea of time travel into the past, to come up with his "chronology protection conjecture" — the notion that some as-yet-unknown law of physics prevents closed timelike curves from happening. But that conjecture is only an educated guess, and until it is supported by hard evidence, we can come to only one conclusion: Time travel is possible.
A party for time travelers
Hawking was skeptical about the feasibility of time travel into the past, not because he had disproved it, but because he was bothered by the logical paradoxes it created. In his chronology protection conjecture, he surmised that physicists would eventually discover a flaw in the theory of closed timelike curves that made them impossible.
In 2009, he came up with an amusing way to test this conjecture. Hawking held a champagne party (shown in his Discovery Channel program), but he only advertised it after it had happened. His reasoning was that, if time machines eventually become practical, someone in the future might read about the party and travel back to attend it. But no one did — Hawking sat through the whole evening on his own. This doesn't prove time travel is impossible, but it does suggest that it never becomes a commonplace occurrence here on Earth.
The arrow of time
One of the distinctive things about time is that it has a direction — from past to future. A cup of hot coffee left at room temperature always cools down; it never heats up. Your cellphone loses battery charge when you use it; it never gains charge. These are examples of entropy , essentially a measure of the amount of "useless" as opposed to "useful" energy. The entropy of a closed system always increases, and it's the key factor determining the arrow of time.
It turns out that entropy is the only thing that makes a distinction between past and future. In other branches of physics, like relativity or quantum theory, time doesn't have a preferred direction. No one knows where time's arrow comes from. It may be that it only applies to large, complex systems, in which case subatomic particles may not experience the arrow of time.
Time travel paradox
If it's possible to travel back into the past — even theoretically — it raises a number of brain-twisting paradoxes — such as the grandfather paradox — that even scientists and philosophers find extremely perplexing.
Killing Hitler
A time traveler might decide to go back and kill him in his infancy. If they succeeded, future history books wouldn't even mention Hitler — so what motivation would the time traveler have for going back in time and killing him?
Killing your grandfather
Instead of killing a young Hitler, you might, by accident, kill one of your own ancestors when they were very young. But then you would never be born, so you couldn't travel back in time to kill them, so you would be born after all, and so on …
A closed loop
Suppose the plans for a time machine suddenly appear from thin air on your desk. You spend a few days building it, then use it to send the plans back to your earlier self. But where did those plans originate? Nowhere — they are just looping round and round in time.
Sign up for the Live Science daily newsletter now
Get the world’s most fascinating discoveries delivered straight to your inbox.
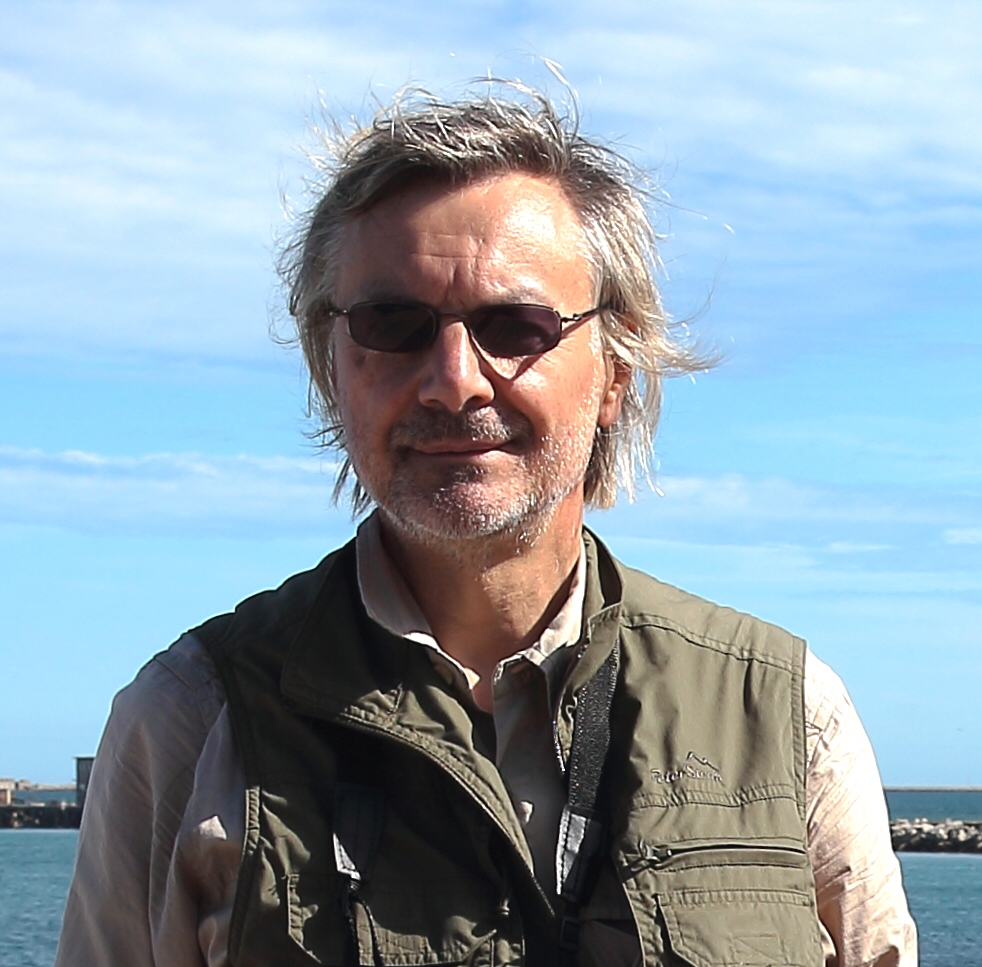
Andrew May holds a Ph.D. in astrophysics from Manchester University, U.K. For 30 years, he worked in the academic, government and private sectors, before becoming a science writer where he has written for Fortean Times, How It Works, All About Space, BBC Science Focus, among others. He has also written a selection of books including Cosmic Impact and Astrobiology: The Search for Life Elsewhere in the Universe, published by Icon Books.
'Severe' geomagnetic storm will bring widespread auroras this weekend after gigantic sunspot spits out 5 solar storms
Gargantuan sunspot 15-Earths wide shoots powerful X-class flare toward Earth, triggering radio blackouts
Stone with 1,600-year-old Irish inscription found in English garden
Most Popular
- 2 New invention transforms any smartphone or TV display into a holographic projector
- 3 1,900-year-old Roman legionary fortress unearthed next to UK cathedral
- 4 Hoard of 17th-century coins hidden during English Civil War unearthed during kitchen renovation
- 5 2,500-year-old Illyrian helmet found in burial mound likely caused 'awe in the enemy'
- 2 30,000 years of history reveals that hard times boost human societies' resilience
- 3 New invention transforms any smartphone or TV display into a holographic projector
- 4 Roman-era skeletons buried in embrace, on top of a horse, weren't lovers, DNA analysis shows
- 5 Gargantuan sunspot 15-Earths wide shoots powerful X-class flare toward Earth, triggering radio blackouts

- May 10, 2024 | Revolutionary Battery Tech Promises Less Charging Time, More Energy Storage
- May 10, 2024 | Quantum Entanglement Unmasked by Entanglement Witnesses
- May 10, 2024 | Innovative Study Reveals How Addiction Hijacks Brain Functions
- May 10, 2024 | New Research Could Lead to More Efficient Televisions, Computer Screens and Lighting
- May 10, 2024 | How Satellite Technology Is Rewriting Tiger Conservation
Exploring the Reality of Time Travel: Science Fact vs. Science Fiction
By Adi Foord, University of Maryland, Baltimore County November 16, 2023
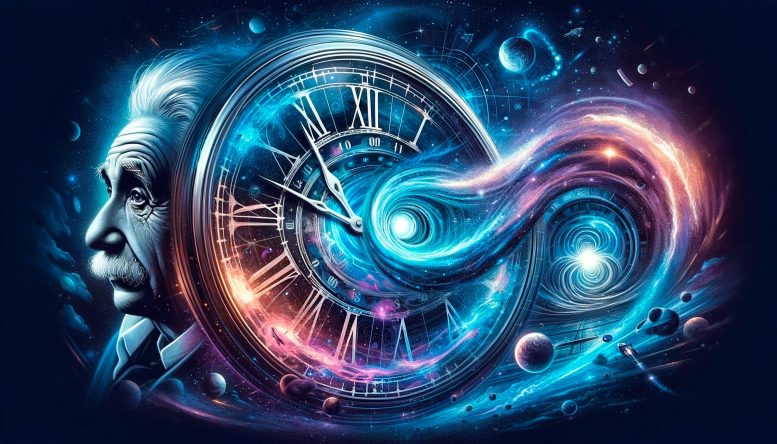
Time travel, a longstanding fascination in science fiction, remains a complex and unresolved concept in science. The second law of thermodynamics suggests time can only move forward, while Einstein’s theory of relativity shows time’s relativity to speed. Theoretical ideas like wormholes offer potential methods, but practical challenges and paradoxes, such as the “grandfather paradox,” complicate the feasibility of actual time travel.
Will it ever be possible for time travel to occur?
Have you ever dreamed of traveling through time, like characters do in science fiction movies? For centuries, the concept of time travel has captivated people’s imaginations. Time travel is the concept of moving between different points in time, just like you move between different places. In movies, you might have seen characters using special machines, magical devices or even hopping into a futuristic car to travel backward or forward in time.
But is this just a fun idea for movies, or could it really happen?
The Science Behind Time Travel
The question of whether time is reversible remains one of the biggest unresolved questions in science. If the universe follows the laws of thermodynamics , it may not be possible. The second law of thermodynamics states that things in the universe can either remain the same or become more disordered over time.
It’s a bit like saying you can’t unscramble eggs once they’ve been cooked. According to this law, the universe can never go back exactly to how it was before. Time can only go forward, like a one-way street.
Time Is Relative
However, physicist Albert Einstein’s theory of special relativity suggests that time passes at different rates for different people. Someone speeding along on a spaceship moving close to the speed of light – 671 million miles per hour! – will experience time slower than a person on Earth.
People have yet to build spaceships that can move at speeds anywhere near as fast as light, but astronauts who visit the International Space Station orbit around the Earth at speeds close to 17,500 mph. Astronaut Scott Kelly has spent 520 days at the International Space Station, and as a result has aged a little more slowly than his twin brother – and fellow astronaut – Mark Kelly. Scott used to be 6 minutes younger than his twin brother. Now, because Scott was traveling so much faster than Mark and for so many days, he is 6 minutes and 5 milliseconds younger .
Theoretical Possibilities and Challenges
Some scientists are exploring other ideas that could theoretically allow time travel. One concept involves wormholes, or hypothetical tunnels in space that could create shortcuts for journeys across the universe. If someone could build a wormhole and then figure out a way to move one end at close to the speed of light – like the hypothetical spaceship mentioned above – the moving end would age more slowly than the stationary end. Someone who entered the moving end and exited the wormhole through the stationary end would come out in their past.
However, wormholes remain theoretical: Scientists have yet to spot one. It also looks like it would be incredibly challenging to send humans through a wormhole space tunnel.
Paradoxes and Failed Dinner Parties
There are also paradoxes associated with time travel. The famous “ grandfather paradox ” is a hypothetical problem that could arise if someone traveled back in time and accidentally prevented their grandparents from meeting. This would create a paradox where you were never born, which raises the question: How could you have traveled back in time in the first place? It’s a mind-boggling puzzle that adds to the mystery of time travel.
Famously, physicist Stephen Hawking tested the possibility of time travel by throwing a dinner party where invitations noting the date, time, and coordinates were not sent out until after it had happened. His hope was that his invitation would be read by someone living in the future, who had capabilities to travel back in time. But no one showed up.
As he pointed out : “The best evidence we have that time travel is not possible, and never will be, is that we have not been invaded by hordes of tourists from the future.”
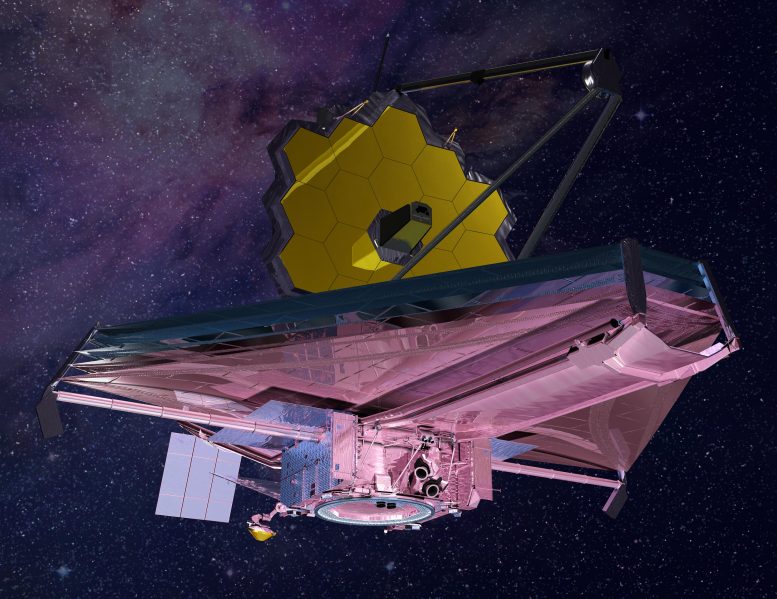
Artist’s rendering of the James Webb Space Telescope. Credit: Northrop Grumman
Telescopes Are Time Machines
Interestingly, astrophysicists armed with powerful telescopes possess a unique form of time travel . As they peer into the vast expanse of the cosmos, they gaze into the past universe. Light from all galaxies and stars takes time to travel, and these beams of light carry information from the distant past. When astrophysicists observe a star or a galaxy through a telescope, they are not seeing it as it is in the present, but as it existed when the light began its journey to Earth millions to billions of years ago.
NASA’s newest space telescope , the James Webb Space Telescope , is peering at galaxies that were formed at the very beginning of the Big Bang , about 13.7 billion years ago.
While we aren’t likely to have time machines like the ones in movies anytime soon, scientists are actively researching and exploring new ideas. But for now, we’ll have to enjoy the idea of time travel in our favorite books, movies, and dreams.
Written by Adi Foord, Assistant Professor of Astronomy and Astrophysics, University of Maryland, Baltimore County.
More on SciTechDaily
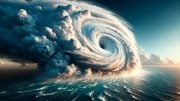
A Significant Surge: How Tropical Cyclones Increase the Cost of Carbon
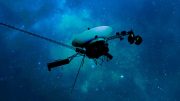
Voyager’s New Horizon: NASA Engineers Tackle Thruster Buildup & Software Glitches
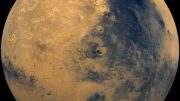
New ScienceCast Video Previews the ‘Opposition of Mars’
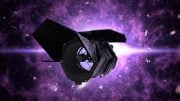
“Our Solar System May Be Unusual”: Rogue Planets Unveiled With NASA’s Roman Space Telescope
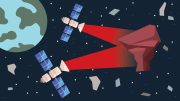
AI-Powered Lasers: A Modern Solution to Space Debris
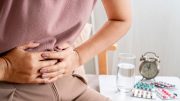
IBS Relief: Common Drug Improves Symptoms of Irritable Bowel Syndrome
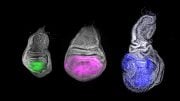
Scientists Discover Chinmo – “The Youth Gene”
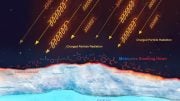
Jupiter’s Moon Europa Could Be Pulling Oxygen Down Below the Ice To Feed Life
8 comments on "exploring the reality of time travel: science fact vs. science fiction".
Until the problem of the second law of thermodynamics(entropy) is solved, the concept of time travel will remain the subject of science fiction. Since this is a basic law of our universe, there is no conceivable way that we know of to do this. The great thing about our knowledge of the universe is that it continues to grow and with that our view of what is possible continues to change. After all, at one time it was believed we could never leave earth!
The 7 planets are soul pollen in the space @ life has been the world has been prepared @ The pollen of the universe is hidden @ Around the axis of the galaxy, the universe is hidden@@ The pollen of the galaxies is a hidden cluster Itis made that we thought about what wisdom is in God’s work and how it is arranged in the form of words.The verse that is made is as follows @@ John, you are in time @ worlds, planets around the axis of the branches of galaxies @@ 8 Prophets, divine prophets, God-aware witnesses @ God’s words of revelation, they are aware @@ 48 What the words of revelation that every prophet has about @ sometimes Sometimes the message of God has become a verbal cliché in the head @@ 62 The message of God was given to every prophet @ The message was made around the power of God @@ 46 The truth of the religions of the cradle of time is said in the world @ Prophets always came for justice in time @@ 62 A warrior became brave in time @ Delaver Ghahrmani Boud Taarani@@ 43 Omar Noah never died, who knows!@ Imransan Is there an unseen world, immortality!?@@ 49 Men’s rights in the sign @ Human rights, the observance of world justice @@ 40 We have God’s love @ A love that is not patched, separation!!!: @@ 39 Take one word from the end of the first and second stanzas to the bottom of these eight verbal verses, and this sentence is made @@ God-aware, the world is born, you know the sign of God @ Agah,the beginning of time, the world, eternity, the world of separation @@ The meaning of this sentence is That God, who before us humans lived on the earth, formed the earth’s crusts with full knowledge, and we humans know the sign of God, which is on the earth, on the continents and countries, and some names have been shown by God for our knowledge since the end of time.In a later video, if I have a lifetime left, I will explain exactly about these poems, God willing @@ The word of the Prophet 17 is the 17th and Muhammad (PBUH) said that the Bedouin Arabs should pray 17 rakats so that theyperform ablution and be clean and not kill and loot.From the caravans, these were all God’s will, and he is good everywhere, in every nation, God does not like evildoers, sinners, and oppressors.Muhammad (PBUH) was God’s last messenger to the Arab people, he was God’s best prophet, and the third verse is because they do not accept Muhammad (PBUH).Some Iranians who were in contact with me, that’s why in the third verse of this surah, he said that his message was given to every prophet, the message is based on divine power, and the word truth, the first two letters of which istruth, is truth, and truth is the 43rd and forty-third word, and the word is time.It is exactly 46, and this song of the Prophets was revealed at the age of 46 ببخشید اگر قبلآ مطالبی فرستادم که به مذاهب مذاهم ارتباط داد شاید به درد شما نمیخورد من در کامنت های بعدی سعی میکنم از نجوم و حیات زمین سخن بگویم این چند بیت را به خارجی انگلیسی که تبدیل کردم معنی آن حیرت انگیز تعغیر کرد گفتم برای شما بفرستم و بداند که این کلام من نبوده کلام خداوند بوده شما نظرتان در مورد خداوند چیست من میگویم خداوند که پدر ومادر انسان بودند قادر به ساختن ما بودند اما آیا آنها قادر به ساختن ستاره ها هم بودند
Your comment has validity to God. But it surely has no place here, it is only fare if the hole comment were in english and has less of a convincing push in a belief a person either believes or not.
It’s too bad physics can’t come to a complete consensus about time, I would like to add some thought about discoveries it has been proven that time travels in only one direction forward, the experiment dealt with light thru glass and how it reacts in the middle and what change happens after light exits the other side, a simple explanation of this experiment. Brings me to theorize and start that time existed before the big bang and is outside of our universes influence, when time is acted on by gravity the ( Form ) of time is changed until the influence no longer has effect, this could go hand in hand with light photons the photon has a influence in the Form that time has. This can not be a observed difference unless we were to see beyond the speed of light. We do agree that physics changes at a subatomic state and also does some strange changing once the speed of light has been exceeded.
The experiment I referred to was posted on IFL in October 2023 headlined ( Solution to complex light problem shows that time can only go Forward ).
One of the problem with travel time is the one people keep forever. And, that is that the earth is always move through space. Matter of fact, the earth is not in the same place that it was 50 years ago. So you will have to move through space as well as time.
Ironically, the only science fiction that seems to handle this is the original story “The Time Machine”.
Time travel is happening now. It has been done since the 1950s. The method satisfies all the requirements. Traveler can’t change the past, but only observe. You can’t go farther back than when the machine was first invented (1950s). There’s one more limitation, you can only observe what the machine was directed. The time machine, the common video camera, and video tape recorder. Now it’s the camera, and file capture computer. Yes, viewing a video tape is effectively going back in time. It’s more than the video, it’s the sound too. There are working versions of smell, and touch which can be recorded too. If you record, and replicate all the senses, you have effectively complete time travel. The most primitive form is the picture. This technology has been around for thousands of years, and is manually intensive. Later many pictures were strung together to make a film. Using a camera to record film was the first example of complete visual time travel (back to when the film was made). Later sound was added, and we have the movies. A way of going back in time that included sight, and sound. Now we have video systems (YouTub) that can play back past events selected by you. Yes, video systems are virtual time travel machines we have now.
How Stellar Cannibalism Illuminates Cosmic Evolution
جزایر فیلیپین دایناسوری که توسط انسان خلق شده در بیش ده ها میلیون سال و بخاطر ریخته شدن دوهزار متر خاک غرق شده بخاطر بالا آمدن آب اقیانوس اما جزایر فیلیپین شبیه دایناسوریست
The address of the above comment on the site about a thief who was trained by a dinosaur bird who was trained by humans tens of millions of years ago and who arrested murderers and robbers.The police were arrested by big birds.don’t the scientists of the world think about this?They were buried in the bed of important cities, they were buried with all the tools and machines, the traces of humans tens of millions of years ago, they had a civilization and a history of hundreds of thousands of years, they built a base underthe earth, from the meteorites that explode from the planets when they hit the sun, and they knew that several thousand meters of soil is poured on the surface of the seas and islands, and they knew that the shapes they made of the islands in thecountry of Papua may go under the ocean, of course, the Philippine islands.The picture is of a baby dinosaur that went under the ocean, but the northern island of Australia, which is Papua, is quite clear.It is a big dinosaur whose tail is towards the east and its mouth is open towards the west.There is the Philippines, but it was more difficult to take the soil to the Philippine islands to create a dinosaur than the island of Papua, that is why the height of the soil in the Philippines is lower, and when the meteorites fell a few kilometerson the surface of the ocean, the image created by humans under the ocean in the shape of a dinosaur is hidden in the American continent The picture is of a bird in the shape of a dinosaur that is flying, and this bird was made to flyby the Indians of the tribe, that bird was talking to people, but its spirit might have heard something from the police because a thief while in the bird’s mouth He was handcuffed by the police and the weapon, which is a machine gun, is fromthe east of the American continent on the coast
The country of Florida is a machine gun.When you continue to New York City, you will reach the mouth of the dinosaur, where a thief is trapped in the mouth of a bird, and the little finger of the police handcuffed the thief’s hand, and a small colt is in the hand ofthe thief, who the police caught in the mouth of the dinosaur.put in the mouth of the bird dinosaur, you can clearly see that the thief fell on the ground and was shot in the head, and it is clear that his forehead was pierced, the bird’s mouth is open in flight, the head of the birdis from the east of the American continent, and a fish is placed in the bird’s mouth in the water of the ocean.The stretched glove of the police, which is in the form of a fist, with a handcuff attached to the left hand of the thief who fell on the ground in the sea and the mouth of the bird, the head of the thief and the killer, is located towards the southwest and west coast of America.All these images were created from the American continent and islands by Humans were created, but they didn’t have enough fuel and time to create more accurate images and meteorites ruined the beauty of the images, but it is clear that all these changes were createdby humans, but you have to consider that two thousand meters of soil from meteorites are fish.And they buried the whales under the beaches, and after a very long time, the bodies of the whales turned into oil under the two thousand meters of soil on the beaches, and on the other hand, the presence of two thousand meters of soil onthe surface of the seas and droughts could not make the created images disappear.
Leave a comment Cancel reply
Email address is optional. If provided, your email will not be published or shared.
Save my name, email, and website in this browser for the next time I comment.
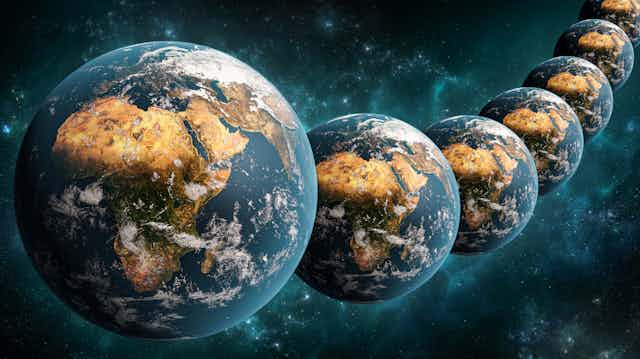
Time travel could be possible, but only with parallel timelines
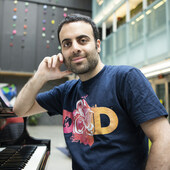
Assistant Professor, Physics, Brock University
Disclosure statement
Barak Shoshany does not work for, consult, own shares in or receive funding from any company or organisation that would benefit from this article, and has disclosed no relevant affiliations beyond their academic appointment.
Brock University provides funding as a member of The Conversation CA-FR.
Brock University provides funding as a member of The Conversation CA.
View all partners
Have you ever made a mistake that you wish you could undo? Correcting past mistakes is one of the reasons we find the concept of time travel so fascinating. As often portrayed in science fiction, with a time machine, nothing is permanent anymore — you can always go back and change it. But is time travel really possible in our universe , or is it just science fiction?
Read more: Curious Kids: is time travel possible for humans?
Our modern understanding of time and causality comes from general relativity . Theoretical physicist Albert Einstein’s theory combines space and time into a single entity — “spacetime” — and provides a remarkably intricate explanation of how they both work, at a level unmatched by any other established theory. This theory has existed for more than 100 years, and has been experimentally verified to extremely high precision, so physicists are fairly certain it provides an accurate description of the causal structure of our universe.
For decades, physicists have been trying to use general relativity to figure out if time travel is possible . It turns out that you can write down equations that describe time travel and are fully compatible and consistent with relativity. But physics is not mathematics, and equations are meaningless if they do not correspond to anything in reality.
Arguments against time travel
There are two main issues which make us think these equations may be unrealistic. The first issue is a practical one: building a time machine seems to require exotic matter , which is matter with negative energy. All the matter we see in our daily lives has positive energy — matter with negative energy is not something you can just find lying around. From quantum mechanics, we know that such matter can theoretically be created, but in too small quantities and for too short times .
However, there is no proof that it is impossible to create exotic matter in sufficient quantities. Furthermore, other equations may be discovered that allow time travel without requiring exotic matter. Therefore, this issue may just be a limitation of our current technology or understanding of quantum mechanics.
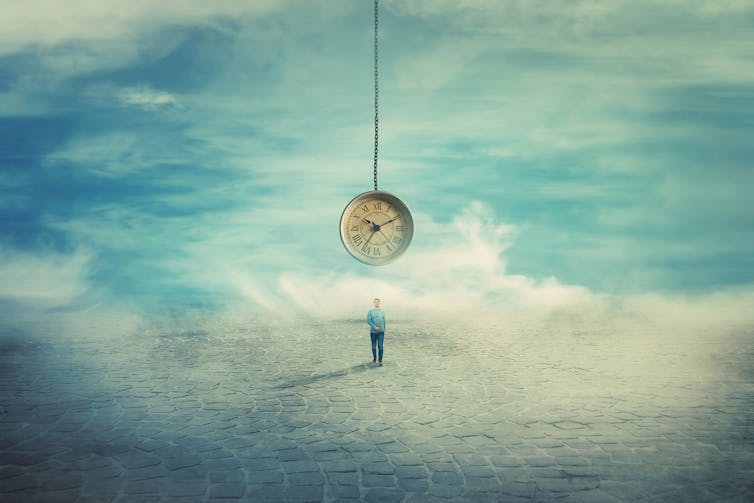
The other main issue is less practical, but more significant: it is the observation that time travel seems to contradict logic, in the form of time travel paradoxes . There are several types of such paradoxes, but the most problematic are consistency paradoxes .
A popular trope in science fiction, consistency paradoxes happen whenever there is a certain event that leads to changing the past, but the change itself prevents this event from happening in the first place.
For example, consider a scenario where I enter my time machine, use it to go back in time five minutes, and destroy the machine as soon as I get to the past. Now that I destroyed the time machine, it would be impossible for me to use it five minutes later.
But if I cannot use the time machine, then I cannot go back in time and destroy it. Therefore, it is not destroyed, so I can go back in time and destroy it. In other words, the time machine is destroyed if and only if it is not destroyed. Since it cannot be both destroyed and not destroyed simultaneously, this scenario is inconsistent and paradoxical.
Eliminating the paradoxes
There’s a common misconception in science fiction that paradoxes can be “created.” Time travellers are usually warned not to make significant changes to the past and to avoid meeting their past selves for this exact reason. Examples of this may be found in many time travel movies, such as the Back to the Future trilogy.
But in physics, a paradox is not an event that can actually happen — it is a purely theoretical concept that points towards an inconsistency in the theory itself. In other words, consistency paradoxes don’t merely imply time travel is a dangerous endeavour, they imply it simply cannot be possible.
This was one of the motivations for theoretical physicist Stephen Hawking to formulate his chronology protection conjecture , which states that time travel should be impossible. However, this conjecture so far remains unproven. Furthermore, the universe would be a much more interesting place if instead of eliminating time travel due to paradoxes, we could just eliminate the paradoxes themselves.
One attempt at resolving time travel paradoxes is theoretical physicist Igor Dmitriyevich Novikov’s self-consistency conjecture , which essentially states that you can travel to the past, but you cannot change it.
According to Novikov, if I tried to destroy my time machine five minutes in the past, I would find that it is impossible to do so. The laws of physics would somehow conspire to preserve consistency.
Introducing multiple histories
But what’s the point of going back in time if you cannot change the past? My recent work, together with my students Jacob Hauser and Jared Wogan, shows that there are time travel paradoxes that Novikov’s conjecture cannot resolve. This takes us back to square one, since if even just one paradox cannot be eliminated, time travel remains logically impossible.
So, is this the final nail in the coffin of time travel? Not quite. We showed that allowing for multiple histories (or in more familiar terms, parallel timelines) can resolve the paradoxes that Novikov’s conjecture cannot. In fact, it can resolve any paradox you throw at it.
The idea is very simple. When I exit the time machine, I exit into a different timeline. In that timeline, I can do whatever I want, including destroying the time machine, without changing anything in the original timeline I came from. Since I cannot destroy the time machine in the original timeline, which is the one I actually used to travel back in time, there is no paradox.
After working on time travel paradoxes for the last three years , I have become increasingly convinced that time travel could be possible, but only if our universe can allow multiple histories to coexist. So, can it?
Quantum mechanics certainly seems to imply so, at least if you subscribe to Everett’s “many-worlds” interpretation , where one history can “split” into multiple histories, one for each possible measurement outcome – for example, whether Schrödinger’s cat is alive or dead, or whether or not I arrived in the past.
But these are just speculations. My students and I are currently working on finding a concrete theory of time travel with multiple histories that is fully compatible with general relativity. Of course, even if we manage to find such a theory, this would not be sufficient to prove that time travel is possible, but it would at least mean that time travel is not ruled out by consistency paradoxes.
Time travel and parallel timelines almost always go hand-in-hand in science fiction, but now we have proof that they must go hand-in-hand in real science as well. General relativity and quantum mechanics tell us that time travel might be possible, but if it is, then multiple histories must also be possible.
- Time travel
- Theoretical physics
- Time machine
- Albert Einstein
- Listen to this article
- Time travel paradox

Events and Communications Coordinator

Assistant Editor - 1 year cadetship

Executive Dean, Faculty of Health

Lecturer/Senior Lecturer, Earth System Science (School of Science)

Sydney Horizon Educators (Identified)
Four ways in which time travel could be possible
Some physicists are convinced that time travel is possible. Here's how we might move through the fourth dimension
- Newsletter sign up Newsletter
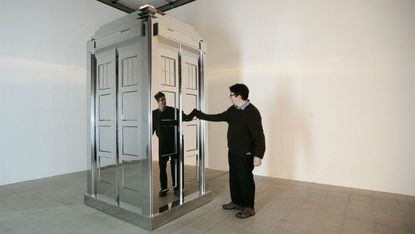
- Time travel 'may be possible', says new research
For as long as there has been science fiction, the concept of time travel has captured the imagination. Though it has long been dismissed as fantasy, physicists have not yet been able to prove or disprove that humans may one day be able to manipulate the fourth dimension.
"Time travel was once considered scientific heresy," writes Professor Stephen Hawking in the Daily Mail . "I used to avoid talking about it for fear of being labelled a crank." However, these days he's far less cautious, admitting he believes human will one day figure out how to travel into the future.
How could time travel be possible?
Subscribe to The Week
Escape your echo chamber. Get the facts behind the news, plus analysis from multiple perspectives.
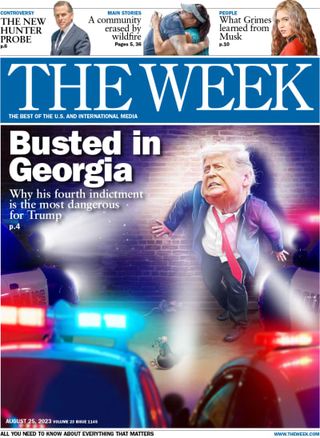
Sign up for The Week's Free Newsletters
From our morning news briefing to a weekly Good News Newsletter, get the best of The Week delivered directly to your inbox.
"The question of time travel features at the interface between two of our most successful yet incompatible physical theories," explains physicist Martin Ringbauer . "Einstein's general relativity and quantum mechanics." Using both of these theories, scientists have suggested several ways they believe time travel could be possible – at least theoretically. These include:
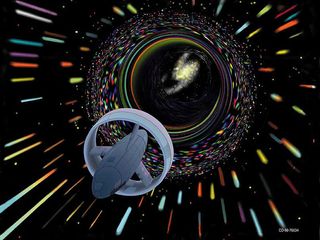
Einstein suggested the theoretical existence of "bridges" through time and space – often referred to as wormholes. His theory has been further developed by numerous physicists including Stephen Hawking and Kip Thorne.
A wormhole would allow an object to travel from one point to another faster than the speed of light, by taking a shortcut through space-time, meaning the object won't break the rule of the so-called universal speed limit — the speed of light — because it will at no point actually travel at a speed faster than light.
"The basic idea if you're very, very optimistic is that if you fiddle with the wormhole openings, you can make it not only a shortcut from a point in space to another point in space, but a shortcut from one moment in time to another moment in time," Professor Brian Greene, a prominent string-theory physicist, told Live Science .
Problem : No wormhole has ever been discovered, and even if it was, it would be far too small for scientists to manipulate for the purposes of time travel – measuring just a billion-trillion-trillionth of a centimetre across. They also pose a significant risk, bringing with them the threat of sudden collapse, high levels of radiation and contact with dangerous exotic matter, Space.com warns.
Wormholes are unstable because of the feedback created by this radiation, explains Hawking. In the same way that excessive feedback between a microphone and a speaker will fry the equipment, a wormhole is damaged by the radiation feedback it generates. "As soon as the wormhole expands, natural radiation will enter it, and end up in a loop," he explains. "So although tiny wormholes do exist, and it may be possible to inflate one someday, it won't last long enough to be of any use as a time machine."
Cosmic strings
Described as one-dimensional "cracks in the universe" and some of the strangest structures observed by cosmologists, cosmic strings could help us navigate through time. "Cosmic strings are either infinite or they're in loops, with no ends", explains J Richard Gott, an astrophysicist at Princeton University. "So they are either like spaghetti or Spaghetti Os."
They are thought to have formed billions of years ago, moments after the Big Bang, and because they contain such large amounts of mass, some scientists believe they could potentially "warp" space-time around them. "The approach of two such strings parallel to each other, will bend space-time so vigorously and in such a particular configuration that [it] might make time travel possible – in theory," according to Live Science.
Problem: Again, cosmic strings only exist only in theory. "This is a project a super civilisation might attempt," says Gott. "It's far beyond what we can do. We're a civilisation that's not even controlling the energy resources of our planet."
Furthermore, in order to travel back in time just twelve months, it would require a cosmic loop that contains half the mass of the entire universe, which we are unlikely to see any time soon.
Supermassive black hole
Described by Professor Hawking as natural time machines, black holes are so dense that they have a dramatic impact on time, slowing it down more than anything else in the universe. If a spaceship were to orbit a black hole, those on board would only experience eight minutes of time for every 16-minute orbit.
"Around and around they'd go, experiencing just half the time of everyone far away from the black hole. The ship and its crew would be travelling through time," he explains. "Imagine they circled the black hole for five years. Ten years would pass elsewhere. When they got home, everyone on Earth would have aged five years more that they had."
Problem: Black holes are more practical than wormholes because they don't present the same paradoxes and won't be destroyed by feedback. "But it's pretty dangerous," concedes Hawking. "It's a long way away and it doesn't even take us very far into the future.
Again, the crew of a ship circling a black hole would need to travel at around the speed of light in order for this plan to work. Physicist Amos Iron at the Technion-Israel Institute of Technology in Haifa, Israel told Space.com of another limitation if one used a machine: it might fall apart before being able to rotate that quickly.
Travelling at the speed of light
Another possibility would be travelling at the speed of light, a constant, finite speed of 186,000 miles per second. In Einstein's special theory of relativity, “time” as a universal concept. Instead, time passes differently for different observers, depending on the observers' motion and - more importantly, speed.
"If you go fast, your clock runs slow relative to people who are still," explains physicist Professor Brian Cox in the Daily Mail . "As you approach the speed of light, your clock runs so slow you could come back 10,000 years in the future."
Problem: According to the laws of physics, nothing can travel as fast as the speed of light – let alone a spaceship. Even the Large Hadron Collider, the strongest particle accelerator in the word, can't make protons move that fast. "If a proton did achieve that speed, it would need infinite energy to go any faster, and we don't have an infinite supply of energy," explains the BBC 's Jennifer Ouellette. Also, the human body would not be able to withstand time travel at all, as travelling at nearly the speed of light would kill you.
This concept is known as time dilation.
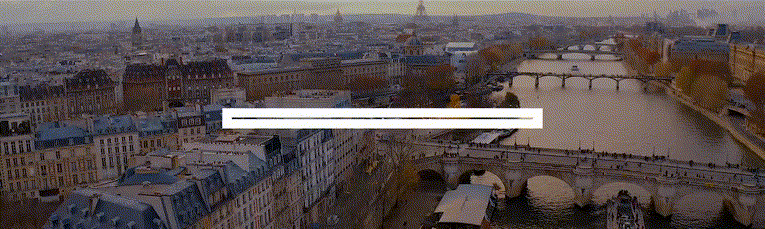
Why time travel may be impossible by any means
Apart from physical problems, several paradoxes stand in the way of time travel. These include the "grandparent paradox", which has long flumoxed physicists and philosophers
As Science Alert explains, a time traveller could in theory prevent his or her grandparents from meeting, "thus preventing the time traveller's birth". This would make it impossible for the time traveller to have set out in the first place and kept the grandparents apart.
However, cosmologists believe they have figured a way around this by suggesting that there is more than one universe in existence – the 'multiverse' model. This allows for every possible version of an event to take place. The Independent 's science editor Steve Connor gives this example: "a woman who goes back in time to murder her own granny can get away with it, because in the universe next door the granny lives to have the daughter who becomes the murderer's mother."
This, and other paradoxes, are situations that "give cosmologists nightmares," writes Hawking. But, "even if it turns out that time travel is impossible, it is important that we understand why it is impossible," he says.
Sign up for Today's Best Articles in your inbox
A free daily email with the biggest news stories of the day – and the best features from TheWeek.com
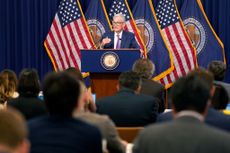
Instant Opinion Opinion, comment and editorials of the day
By Harold Maass, The Week US Published 10 May 24
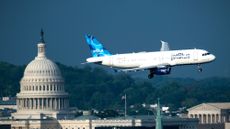
Speed Read The legislation will require airlines to refund customers for flight delays
By Peter Weber, The Week US Published 10 May 24
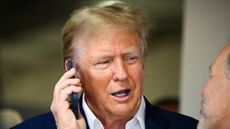
Speed Read The former president promised to reverse Biden's environmental regulations if elected
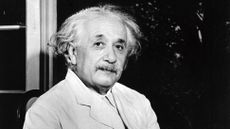
In Depth Einstein’s theory remains ‘most important in modern physics’
By James Ashford Last updated 15 May 20
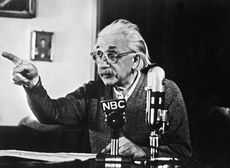
Speed Read Famous note penned by theoretical physicist goes up for auction today
By The Week Staff Last updated 4 December 18
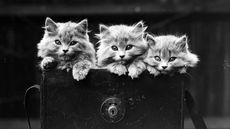
In Depth The thought experiment lives on – or does it? What Schrodinger's cat tells us about quantum physics
By The Week Staff Published 16 August 17
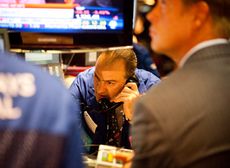
Daily Briefing
By The Week Staff Published 23 September 11
- Contact Future's experts
- Terms and Conditions
- Privacy Policy
- Cookie Policy
- Advertise With Us
The Week is part of Future plc, an international media group and leading digital publisher. Visit our corporate site . © Future US, Inc. Full 7th Floor, 130 West 42nd Street, New York, NY 10036.
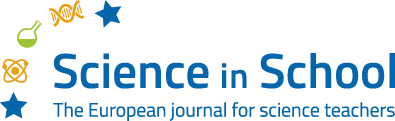
Science in School
Time travel: science fact or science fiction understand article.
Author(s): Jim Al-Khalili
Do you believe that time travel has no place in a serious science lesson? Jim Al-Khalili from the University of Surrey, UK, disagrees. He shows how the topic of time travel introduces some of the ideas behind Einstein’s theories of relativity.
When physicists want to get people excited about their subject, they usually turn to cosmology or particle physics. There is always going to be something exciting to say about space or the search for new particles at the Large Hadron Collider (for a discussion of the LHC, see Landua & Rau, 2008 , and Landua, 2008 ). Of course, it is far more challenging to make electromagnetism or thermodynamics sound exciting.
So is introducing a subject like time travel a little like giving in to science fiction? I would argue not. In fact, I think it is an excellent way of getting across some of the basic ideas behind Einstein’s theories of relativity (yes, there are two of them). One can begin by asking the simple yet emotive question: is time travel really possible? Anyone who has watched a movie such as The Terminator or is a fan of Dr Who may worry that the concept of time travel, while great fun to contemplate, is just nonsense with no place in real science. However, not only do the laws of physics allow for time travel, but it has also been proven possible in many experiments.
Of course I should qualify this bold statement by pointing out that only time travel into the future has actually been achieved. Time travel into the past is much more difficult, and is probably impossible. What is so fascinating, though, is that it cannot be ruled out yet. My aim in this article is to explain the difference between these two directions of time travel and to show how relativity theory forced physicists to abandon common-sense notions about the nature of time itself.
Isaac Newton: the common sense approach?
Until Isaac Newton completed his work on the laws of motion in 1687, the definition of time had been considered to be the domain of philosophy rather than science. However, when Newton described how objects move under the influence of forces, time was an integral part of his mathematical description of reality, since all movement and change require the notion of time to make sense. Newton viewed time as absolute and relentless; he described it as existing entirely outside of space and independent of all processes that occur within space. This is still the view that most of us have: we think of time as flowing at a constant rate, as though there were an imaginary cosmic clock that marks off the seconds, hours and years regardless of our feelings about the passage of time. We have no influence on its rate of flow and cannot make it speed up or slow down. We feel we know what time is – but no one really does. The best definitions we have are rather silly, like saying ‘time is nature’s way of stopping everything happening at once!’ or ‘time flows by at a constant rate of one second every second’. What on earth does that mean anyway?
Was Newton right? Does such absolute time really exist? Albert Einstein showed that it doesn’t.
Albert Einstein: a revolutionary
In 1905, Einstein discovered, through his study of the nature of light, that time and space are not independent but are intimately linked. His ideas became known as the special theory of relativity, which heralded a revolution in physics. It showed how and why the old notions of space and time had to be replaced with a new and unfamiliar set of concepts. Basically, relativity theory unifies time with the three dimensions of space into something called space-time. This is where the idea of time as the fourth dimension comes from.
In 1915, ten years after his work on special relativity, Einstein completed his theory of gravity, known as the general theory of relativity. Widely regarded as the most beautiful scientific theory ever discovered, it describes how the gravitational effects of matter affect space-time. This led to many exciting predictions that were subsequently shown to be correct, such as the birth of the Universe in the Big Bang and the existence of black holes.
But let us return to the topic of special relativity. Einstein showed that for anything (or anyone) travelling at speeds approaching that of light – an impressive three hundred thousand kilometres per second – time literally runs more slowly. The closer to the speed of light that a clock moves, the slower it will tick as seen by observers watching it zoom past. Nowadays, the slowing down of time is confirmed routinely in particle accelerators, such as the CERN facility in Switzerland w1 . Many physics students get a chance to see this effect in the laboratory by observing a certain type of sub-atomic particle called a muon (pronounced ‘mew-on’) w2 .
Let us consider a simple example with numbers. A sprinter runs 100 metres in exactly ten seconds, according to the reliable and highly accurate timekeeping of the judges. Had he, however, carried his own very accurate stopwatch along with him, then, due to time slowing down very slightly for him, his watch would show a time of 9.999999999995 seconds. Of course, this is so close to ten seconds that we would never know the difference. However, scientists routinely need to measure times with this sort of accuracy. The difference between the runner’s and the judges’ watches is just five picoseconds; it is such a small time difference because the athlete is moving so much slower than light.
This is actually quite a subtle concept. If people know anything about the theory of relativity, it tends to be its insistence that all motion is relative. So why is it the sprinter’s watch that runs slower, hence recording the shorter time? If all motion is relative, then we should be able to argue that it is in fact the track that is moving relative to the sprinter. So it should be the judges’ trackside watches that run slower. This is true, but in reality the situation is not completely symmetrical. For one thing, the sprinter has to accelerate and decelerate and this change in speed affects the rate at which his time goes by, relative to that of the judges. Another way of understanding why the sprinter’s stopwatch reads a faster time is that, for him, the distance he has to run is in fact slightly less than 100 metres. This is another consequence of the theory of relativity: that distances are shortened when you move very fast.
High-speed motion: time travel to the future
As this is beginning to sound somewhat strange, we might as well explore how it all links up with time travel. The idea of time slowing down gives us, quite literally, a means of time travel into the future. If you were to travel around our galaxy in a rocket, at close to the speed of light for, say, four years, you would get quite a shock when you returned home to Earth. If your onboard calendar said you left in January 2005 and returned in January 2009, then depending on your exact speed and how twisted your path was through the stars, you might find that on Earth, the year was 2045 and everyone had aged 40 years! They would be equally shocked to see how young you still looked considering how long you had, according to them, been away.
Inside the rocket, four years would have elapsed while Earth-bound clocks counted off 40 years. This means that you would have, for all intents and purposes, leapt 36 years into the future.
This effect has been checked and confirmed many times in different experiments to extremely high degrees of accuracy. In 1971, J. C. Hafele and Richard E. Keating placed four highly accurate atomic clocks on a jet aircraft and flew them eastwards around Earth. After the jet returned, the onboard clocks were compared with reference atomic clocks at the US Naval Observatory: the travelling clocks were a tiny fraction of a second behind the reference clocks ( Hafele & Keating, 1972a , 1972b ) w3 . Even though the jet had a ground speed of up to a thousand kilometres per hour, the speed of light is a further million times greater than this, hence the very small and rather unimpressive difference between the two groups of clocks. Nevertheless, that difference is real and the clocks are so accurate that we do not doubt their readings or the conclusions we draw from them.
Time travel to the past?
Time travel to the past, it turns out, is much more difficult. To many people, it might come as a surprise that travelling forward in time is easier than travelling backward. If anything, you might think that the notion of travelling into the future is the more ridiculous. The past may well be inaccessible, but at least it is out there in some sense: it has happened. The future on the other hand, has yet to happen. How can we visit a time that has not yet happened? However, time travel to the future by high-speed motion does not require the future to be already ‘out there’ waiting for us. What it means is that we move out of everyone else’s time frame and into one in which time moves more slowly. While we are in this state, time outside ticks by more quickly and the future unfolds at high speed. When we rejoin our original time frame, we will have reached the future more quickly than everyone else.
On the other hand, there are many mind-boggling examples of how ridiculous things would be if time travel to the past were possible. For example, what if you were to go back in time, to last year for example, and kill your younger self. What would happen then? Would you simply pop out of existence as the younger you slumps to the ground? And if you died last year, who would have killed you? I know this is a bit morbid, but it is a well-known paradox. Think about it. It seems you cannot kill your younger self because you must survive the assassination attempt to become the assassin. The thing to remember about time travel to the past is that you are allowed to meddle with history as long as things still turn out the way they do. You cannot change the past.
In principle, there would be two ways of going back to the past. One is by going backwards through time, during which the hands of your watch would move anticlockwise. This would require faster-than-light speeds which relativity theory tells us are impossible, and so is not the sort of time travel I am discussing here. The other way is by travelling what appears to you to be forward in time (your watch runs forwards) but by moving along a warped path through space-time that takes you back to your past (like looping the loop on a roller coaster). Such a loop is known in physics as a closed time-like curve and has been the subject of intense theoretical research during the past decade. Perhaps surprisingly, it has been known for half a century that Einstein’s equations of general relativity allow such closed time-like curves: the American mathematician Kurt Gödel showed in 1949 that this type of time travel into the past was theoretically possible.
So what is all the fuss about? Time travel to the future has been done and time travel to the past, while difficult, has not been ruled out by theory. What are we waiting for? Why haven’t we built a time machine yet? The problem is that, apart from the fact that closed time-like curves in space-time are exceedingly difficult to create, we do not really understand them theoretically. As things stand at the beginning of the 21st century, general relativity tells us that we cannot rule out time travel, but many physicists are hoping that a better understanding of the mathematics involved will eventually lead to the conclusion that time loops are impossible.
At the moment, we cannot rule out the possibility that a naturally occurring time machine exists somewhere in the Universe. It is theoretically possible for space-time to be so warped in the presence of a very strong gravitational field that, under certain special conditions, a time loop is created. If we stumbled across such an entity, known as a wormhole, during future space travel it might provide us with a permanent link to the past.
For now, the best way to rule out the existence of time loops is to ask where all the time travellers from the future are. If future generations ever succeeded in building a time machine then surely there would be many people wanting to visit the 21st century and we should see these visitors among us today. So just to keep the debate alive, below are five possible reasons why we should not expect to see any time travellers:
- Time travel to the past is forbidden by some as-yet-undiscovered laws of physics. Physicists hope to discover a new theory that goes beyond general relativity and which explains why time loops are forbidden. We already have two possible candidates for such a theory, known as superstring theory and membrane theory. But neither is properly understood yet.
- There are no naturally occurring time machines, such as wormholes, so the only way to travel back in time is to build one. But it turns out that this would only take us as far back as the moment it was switched on (because that would be the earliest moment in time that could be accessed). So we cannot see any time travellers from the future because time machines have not been invented yet.
- Naturally occurring time machines exist and people do use them to travel back to the 21st century, but – an idea taken seriously by many theoretical physicists – our universe is just one of an infinite number of parallel universes. Thus time travel to the past slides the traveller into a parallel world. There are so many of these that our universe is just not one of the lucky few that have been visited. If you are not convinced by these reasons, then I might interest you in a couple of more mundane possibilities:
- Expecting to see time travellers among us presupposes that they would want to visit this century. Maybe for them, there are much nicer and safer periods to visit.
- Time travellers from the future are among us but they keep a low profile!
If I were a betting man I would say that time travel to the past will soon be shown to be impossible even in theory. Getting to the future, on the other hand, just requires us to build a fast enough rocket. Beware, though, that if you reach the future, there is no coming back.
- Hafele JC, Keating RE (1972a) Around-the-world atomic clocks: predicted relativistic time gains. Science 177(4044) : 166-167. doi: 10.1126/science.177.4044.166
- Hafele JC, Keating RE (1972b) Around-the-world atomic clocks: observed relativistic time gains. Science 177(4044) : 168-170. doi: 10.1126/science.177.4044.168
- Landua R (2008) The LHC: a look inside . Science in School 10 : 34-45.
Web References
- w1 – For more information about CERN, the world’s largest particle physics laboratory, see: www.cern.ch
- www.teachspin.com/instruments/muon_physics/experiments.shtml
- www.jlab.org/~cecire/muonlife.html
- www.physics.smu.edu/~coan/outreach/overview.html
- w3 – For more information about the Hafele-Keating experiment, see: http://en.wikipedia.org/wiki/Hafele-Keating_experiment
On relativity and time travel
Al-Khalili J (1999) BlackHoles, Wormholes and Time Machines . London, UK: Taylor and Francis
On time travel
Davies P (2002) How to Build a Time Machine . London, UK: Penguin
On relativity
Epstein LC (1981) Relativity Visualised . San Francisco, CA, USA: Insight Press Mermin ND (1989) Space and Time in Special Relativity. Prospect Heights, IL, USA: Waveland Press
Stannard R (1989) The Time and Space of Uncle Albert . London, UK: Faber and Faber
On string theory
Greene B (2000) The Elegant Universe: Superstrings, Hidden Dimensions and the Quest for the Ultimate Theory . New York, NY, USA: Vintage
Jim Al-Khalili is a physics professor and the Professor of the Public Engagement in Science at the University of Surrey, where he has taught a course on relativity to undergraduates for the past 12 years. He appears regularly on TV and radio.
What do we know about time travel? Is it possible to travel to the future,or to the past? To what extent has it been tested, and what were the results? This article gives short but very exciting answers to these questions.
The article is very useful to introduce relativity and associated topics of modern physics to the students. Teachers can use it to discuss issues such as the nature of time and its meaning, or the exciting topic of time travel. It allows for a combination of physics with philosophy.
Taking the article as a starting point, teachers can then discuss further problems of time travel, such as the difficulty of accelerating a 70 kg human to the speed of light.
Alessandro Iscra, Italy
Download this article as a PDF
Share this article
Subscribe to our newsletter.


- Table of Contents
- Random Entry
- Chronological
- Editorial Information
- About the SEP
- Editorial Board
- How to Cite the SEP
- Special Characters
- Advanced Tools
- Support the SEP
- PDFs for SEP Friends
- Make a Donation
- SEPIA for Libraries
- Entry Contents
Bibliography
Academic tools.
- Friends PDF Preview
- Author and Citation Info
- Back to Top
Time Travel and Modern Physics
Time travel has been a staple of science fiction. With the advent of general relativity it has been entertained by serious physicists. But, especially in the philosophy literature, there have been arguments that time travel is inherently paradoxical. The most famous paradox is the grandfather paradox: you travel back in time and kill your grandfather, thereby preventing your own existence. To avoid inconsistency some circumstance will have to occur which makes you fail in this attempt to kill your grandfather. Doesn’t this require some implausible constraint on otherwise unrelated circumstances? We examine such worries in the context of modern physics.
1. Paradoxes Lost?
2. topology and constraints, 3. the general possibility of time travel in general relativity, 4. two toy models, 5. slightly more realistic models of time travel, 6. the possibility of time travel redux, 7. even if there are constraints, so what, 8. computational models, 9. quantum mechanics to the rescue, 10. conclusions, other internet resources, related entries.
- Supplement: Remarks and Limitations on the Toy Models
Modern physics strips away many aspects of the manifest image of time. Time as it appears in the equations of classical mechanics has no need for a distinguished present moment, for example. Relativity theory leads to even sharper contrasts. It replaces absolute simultaneity, according to which it is possible to unambiguously determine the time order of distant events, with relative simultaneity: extending an “instant of time” throughout space is not unique, but depends on the state of motion of an observer. More dramatically, in general relativity the mathematical properties of time (or better, of spacetime)—its topology and geometry—depend upon how matter is arranged rather than being fixed once and for all. So physics can be, and indeed has to be, formulated without treating time as a universal, fixed background structure. Since general relativity represents gravity through spacetime geometry, the allowed geometries must be as varied as the ways in which matter can be arranged. Alongside geometrical models used to describe the solar system, black holes, and much else, the scope of variation extends to include some exotic structures unlike anything astrophysicists have observed. In particular, there are spacetime geometries with curves that loop back on themselves: closed timelike curves (CTCs), which describe the possible trajectory of an observer who returns exactly back to their earlier state—without any funny business, such as going faster than the speed of light. These geometries satisfy the relevant physical laws, the equations of general relativity, and in that sense time travel is physically possible.
Yet circular time generates paradoxes, familiar from science fiction stories featuring time travel: [ 1 ]
- Consistency: Kurt plans to murder his own grandfather Adolph, by traveling along a CTC to an appropriate moment in the past. He is an able marksman, and waits until he has a clear shot at grandpa. Normally he would not miss. Yet if he succeeds, there is no way that he will then exist to plan and carry out the mission. Kurt pulls the trigger: what can happen?
- Underdetermination: Suppose that Kurt first travels back in order to give his earlier self a copy of How to Build a Time Machine. This is the same book that allows him to build a time machine, which he then carries with him on his journey to the past. Who wrote the book?
- Easy Knowledge: A fan of classical music enhances their computer with a circuit that exploits a CTC. This machine efficiently solves problems at a higher level of computational complexity than conventional computers, leading (among other things) to finding the smallest circuits that can generate Bach’s oeuvre—and to compose new pieces in the same style. Such easy knowledge is at odds with our understanding of our epistemic predicament. (This third paradox has not drawn as much attention.)
The first two paradoxes were once routinely taken to show that solutions with CTCs should be rejected—with charges varying from violating logic, to being “physically unreasonable”, to undermining the notion of free will. Closer analysis of the paradoxes has largely reversed this consensus. Physicists have discovered many solutions with CTCs and have explored their properties in pursuing foundational questions, such as whether physics is compatible with the idea of objective temporal passage (starting with Gödel 1949). Philosophers have also used time travel scenarios to probe questions about, among other things, causation, modality, free will, and identity (see, e.g., Earman 1972 and Lewis’s seminal 1976 paper).
We begin below with Consistency , turning to the other paradoxes in later sections. A standard, stone-walling response is to insist that the past cannot be changed, as a matter of logic, even by a time traveler (e.g., Gödel 1949, Clarke 1977, Horwich 1987). Adolph cannot both die and survive, as a matter of logic, so any scheme to alter the past must fail. In many of the best time travel fictions, the actions of a time traveler are constrained in novel and unexpected ways. Attempts to change the past fail, and they fail, often tragically, in just such a way that they set the stage for the time traveler’s self-defeating journey. The first question is whether there is an analog of the consistent story when it comes to physics in the presence of CTCs. As we will see, there is a remarkable general argument establishing the existence of consistent solutions. Yet a second question persists: why can’t time-traveling Kurt kill his own grandfather? Doesn’t the necessity of failures to change the past put unusual and unexpected constraints on time travelers, or objects that move along CTCs? The same argument shows that there are in fact no constraints imposed by the existence of CTCs, in some cases. After discussing this line of argument, we will turn to the palatability and further implications of such constraints if they are required, and then turn to the implications of quantum mechanics.
Wheeler and Feynman (1949) were the first to claim that the fact that nature is continuous could be used to argue that causal influences from later events to earlier events, as are made possible by time travel, will not lead to paradox without the need for any constraints. Maudlin (1990) showed how to make their argument precise and more general, and argued that nonetheless it was not completely general.
Imagine the following set-up. We start off having a camera with a black and white film ready to take a picture of whatever comes out of the time machine. An object, in fact a developed film, comes out of the time machine. We photograph it, and develop the film. The developed film is subsequently put in the time machine, and set to come out of the time machine at the time the picture is taken. This surely will create a paradox: the developed film will have the opposite distribution of black, white, and shades of gray, from the object that comes out of the time machine. For developed black and white films (i.e., negatives) have the opposite shades of gray from the objects they are pictures of. But since the object that comes out of the time machine is the developed film itself it we surely have a paradox.
However, it does not take much thought to realize that there is no paradox here. What will happen is that a uniformly gray picture will emerge, which produces a developed film that has exactly the same uniform shade of gray. No matter what the sensitivity of the film is, as long as the dependence of the brightness of the developed film depends in a continuous manner on the brightness of the object being photographed, there will be a shade of gray that, when photographed, will produce exactly the same shade of gray on the developed film. This is the essence of Wheeler and Feynman’s idea. Let us first be a bit more precise and then a bit more general.
For simplicity let us suppose that the film is always a uniform shade of gray (i.e., at any time the shade of gray does not vary by location on the film). The possible shades of gray of the film can then be represented by the (real) numbers from 0, representing pure black, to 1, representing pure white.
Let us now distinguish various stages in the chronological order of the life of the film. In stage \(S_1\) the film is young; it has just been placed in the camera and is ready to be exposed. It is then exposed to the object that comes out of the time machine. (That object in fact is a later stage of the film itself). By the time we come to stage \(S_2\) of the life of the film, it has been developed and is about to enter the time machine. Stage \(S_3\) occurs just after it exits the time machine and just before it is photographed. Stage \(S_4\) occurs after it has been photographed and before it starts fading away. Let us assume that the film starts out in stage \(S_1\) in some uniform shade of gray, and that the only significant change in the shade of gray of the film occurs between stages \(S_1\) and \(S_2\). During that period it acquires a shade of gray that depends on the shade of gray of the object that was photographed. In other words, the shade of gray that the film acquires at stage \(S_2\) depends on the shade of gray it has at stage \(S_3\). The influence of the shade of gray of the film at stage \(S_3\), on the shade of gray of the film at stage \(S_2\), can be represented as a mapping, or function, from the real numbers between 0 and 1 (inclusive), to the real numbers between 0 and 1 (inclusive). Let us suppose that the process of photography is such that if one imagines varying the shade of gray of an object in a smooth, continuous manner then the shade of gray of the developed picture of that object will also vary in a smooth, continuous manner. This implies that the function in question will be a continuous function. Now any continuous function from the real numbers between 0 and 1 (inclusive) to the real numbers between 0 and 1 (inclusive) must map at least one number to itself. One can quickly convince oneself of this by graphing such functions. For one will quickly see that any continuous function \(f\) from \([0,1]\) to \([0,1]\) must intersect the line \(x=y\) somewhere, and thus there must be at least one point \(x\) such that \(f(x)=x\). Such points are called fixed points of the function. Now let us think about what such a fixed point represents. It represents a shade of gray such that, when photographed, it will produce a developed film with exactly that same shade of gray. The existence of such a fixed point implies a solution to the apparent paradox.
Let us now be more general and allow color photography. One can represent each possible color of an object (of uniform color) by the proportions of blue, green and red that make up that color. (This is why television screens can produce all possible colors.) Thus one can represent all possible colors of an object by three points on three orthogonal lines \(x, y\) and \(z\), that is to say, by a point in a three-dimensional cube. This cube is also known as the “Cartesian product” of the three line segments. Now, one can also show that any continuous map from such a cube to itself must have at least one fixed point. So color photography can not be used to create time travel paradoxes either!
Even more generally, consider some system \(P\) which, as in the above example, has the following life. It starts in some state \(S_1\), it interacts with an object that comes out of a time machine (which happens to be its older self), it travels back in time, it interacts with some object (which happens to be its younger self), and finally it grows old and dies. Let us assume that the set of possible states of \(P\) can be represented by a Cartesian product of \(n\) closed intervals of the reals, i.e., let us assume that the topology of the state-space of \(P\) is isomorphic to a finite Cartesian product of closed intervals of the reals. Let us further assume that the development of \(P\) in time, and the dependence of that development on the state of objects that it interacts with, is continuous. Then, by a well-known fixed point theorem in topology (see, e.g., Hocking & Young 1961: 273), no matter what the nature of the interaction is, and no matter what the initial state of the object is, there will be at least one state \(S_3\) of the older system (as it emerges from the time travel machine) that will influence the initial state \(S_1\) of the younger system (when it encounters the older system) so that, as the younger system becomes older, it develops exactly into state \(S_3\). Thus without imposing any constraints on the initial state \(S_1\) of the system \(P\), we have shown that there will always be perfectly ordinary, non-paradoxical, solutions, in which everything that happens, happens according to the usual laws of development. Of course, there is looped causation, hence presumably also looped explanation, but what do you expect if there is looped time?
Unfortunately, for the fan of time travel, a little reflection suggests that there are systems for which the needed fixed point theorem does not hold. Imagine, for instance, that we have a dial that can only rotate in a plane. We are going to put the dial in the time machine. Indeed we have decided that if we see the later stage of the dial come out of the time machine set at angle \(x\), then we will set the dial to \(x+90\), and throw it into the time machine. Now it seems we have a paradox, since the mapping that consists of a rotation of all points in a circular state-space by 90 degrees does not have a fixed point. And why wouldn’t some state-spaces have the topology of a circle?
However, we have so far not used another continuity assumption which is also a reasonable assumption. So far we have only made the following demand: the state the dial is in at stage \(S_2\) must be a continuous function of the state of the dial at stage \(S_3\). But, the state of the dial at stage \(S_2\) is arrived at by taking the state of the dial at stage \(S_1\), and rotating it over some angle. It is not merely the case that the effect of the interaction, namely the state of the dial at stage \(S_2\), should be a continuous function of the cause, namely the state of the dial at stage \(S_3\). It is additionally the case that path taken to get there, the way the dial is rotated between stages \(S_1\) and \(S_2\) must be a continuous function of the state at stage \(S_3\). And, rather surprisingly, it turns out that this can not be done. Let us illustrate what the problem is before going to a more general demonstration that there must be a fixed point solution in the dial case.
Forget time travel for the moment. Suppose that you and I each have a watch with a single dial neither of which is running. My watch is set at 12. You are going to announce what your watch is set at. My task is going to be to adjust my watch to yours no matter what announcement you make. And my actions should have a continuous (single valued) dependence on the time that you announce. Surprisingly, this is not possible! For instance, suppose that if you announce “12”, then I achieve that setting on my watch by doing nothing. Now imagine slowly and continuously increasing the announced times, starting at 12. By continuity, I must achieve each of those settings by rotating my dial to the right. If at some point I switch and achieve the announced goal by a rotation of my dial to the left, I will have introduced a discontinuity in my actions, a discontinuity in the actions that I take as a function of the announced angle. So I will be forced, by continuity, to achieve every announcement by rotating the dial to the right. But, this rotation to the right will have to be abruptly discontinued as the announcements grow larger and I eventually approach 12 again, since I achieved 12 by not rotating the dial at all. So, there will be a discontinuity at 12 at the latest. In general, continuity of my actions as a function of announced times can not be maintained throughout if I am to be able to replicate all possible settings. Another way to see the problem is that one can similarly reason that, as one starts with 12, and imagines continuously making the announced times earlier, one will be forced, by continuity, to achieve the announced times by rotating the dial to the left. But the conclusions drawn from the assumption of continuous increases and the assumption of continuous decreases are inconsistent. So we have an inconsistency following from the assumption of continuity and the assumption that I always manage to set my watch to your watch. So, a dial developing according to a continuous dynamics from a given initial state, can not be set up so as to react to a second dial, with which it interacts, in such a way that it is guaranteed to always end up set at the same angle as the second dial. Similarly, it can not be set up so that it is guaranteed to always end up set at 90 degrees to the setting of the second dial. All of this has nothing to do with time travel. However, the impossibility of such set ups is what prevents us from enacting the rotation by 90 degrees that would create paradox in the time travel setting.
Let us now give the positive result that with such dials there will always be fixed point solutions, as long as the dynamics is continuous. Let us call the state of the dial before it interacts with its older self the initial state of the dial. And let us call the state of the dial after it emerges from the time machine the final state of the dial. There is also an intermediate state of the dial, after it interacts with its older self and before it is put into the time machine. We can represent the initial or intermediate states of the dial, before it goes into the time machine, as an angle \(x\) in the horizontal plane and the final state of the dial, after it comes out of the time machine, as an angle \(y\) in the vertical plane. All possible \(\langle x,y\rangle\) pairs can thus be visualized as a torus with each \(x\) value picking out a vertical circular cross-section and each \(y\) picking out a point on that cross-section. See figure 1 .
Figure 1 [An extended description of figure 1 is in the supplement.]
Suppose that the dial starts at angle \(i\) which picks out vertical circle \(I\) on the torus. The initial angle \(i\) that the dial is at before it encounters its older self, and the set of all possible final angles that the dial can have when it emerges from the time machine is represented by the circle \(I\) on the torus (see figure 1 ). Given any possible angle of the emerging dial, the dial initially at angle \(i\) will develop to some other angle. One can picture this development by rotating each point on \(I\) in the horizontal direction by the relevant amount. Since the rotation has to depend continuously on the angle of the emerging dial, circle \(I\) during this development will deform into some loop \(L\) on the torus. Loop \(L\) thus represents all possible intermediate angles \(x\) that the dial is at when it is thrown into the time machine, given that it started at angle \(i\) and then encountered a dial (its older self) which was at angle \(y\) when it emerged from the time machine. We therefore have consistency if \(x=y\) for some \(x\) and \(y\) on loop \(L\). Now, let loop \(C\) be the loop which consists of all the points on the torus for which \(x=y\). Ring \(I\) intersects \(C\) at point \(\langle i,i\rangle\). Obviously any continuous deformation of \(I\) must still intersect \(C\) somewhere. So \(L\) must intersect \(C\) somewhere, say at \(\langle j,j\rangle\). But that means that no matter how the development of the dial starting at \(I\) depends on the angle of the emerging dial, there will be some angle for the emerging dial such that the dial will develop exactly into that angle (by the time it enters the time machine) under the influence of that emerging dial. This is so no matter what angle one starts with, and no matter how the development depends on the angle of the emerging dial. Thus even for a circular state-space there are no constraints needed other than continuity.
Unfortunately there are state-spaces that escape even this argument. Consider for instance a pointer that can be set to all values between 0 and 1, where 0 and 1 are not possible values. That is, suppose that we have a state-space that is isomorphic to an open set of real numbers. Now suppose that we have a machine that sets the pointer to half the value that the pointer is set at when it emerges from the time machine.
Figure 2 [An extended description of figure 2 is in the supplement.]
Suppose the pointer starts at value \(I\). As before we can represent the combination of this initial position and all possible final positions by the line \(I\). Under the influence of the pointer coming out of the time machine the pointer value will develop to a value that equals half the value of the final value that it encountered. We can represent this development as the continuous deformation of line \(I\) into line \(L\), which is indicated by the arrows in figure 2 . This development is fully continuous. Points \(\langle x,y\rangle\) on line \(I\) represent the initial position \(x=I\) of the (young) pointer, and the position \(y\) of the older pointer as it emerges from the time machine. Points \(\langle x,y\rangle\) on line \(L\) represent the position \(x\) that the younger pointer should develop into, given that it encountered the older pointer emerging from the time machine set at position \(y\). Since the pointer is designed to develop to half the value of the pointer that it encounters, the line \(L\) corresponds to \(x=1/2 y\). We have consistency if there is some point such that it develops into that point, if it encounters that point. Thus, we have consistency if there is some point \(\langle x,y\rangle\) on line \(L\) such that \(x=y\). However, there is no such point: lines \(L\) and \(C\) do not intersect. Thus there is no consistent solution, despite the fact that the dynamics is fully continuous.
Of course if 0 were a possible value, \(L\) and \(C\) would intersect at 0. This is surprising and strange: adding one point to the set of possible values of a quantity here makes the difference between paradox and peace. One might be tempted to just add the extra point to the state-space in order to avoid problems. After all, one might say, surely no measurements could ever tell us whether the set of possible values includes that exact point or not. Unfortunately there can be good theoretical reasons for supposing that some quantity has a state-space that is open: the set of all possible speeds of massive objects in special relativity surely is an open set, since it includes all speeds up to, but not including, the speed of light. Quantities that have possible values that are not bounded also lead to counter examples to the presented fixed point argument. And it is not obvious to us why one should exclude such possibilities. So the argument that no constraints are needed is not fully general.
An interesting question of course is: exactly for which state-spaces must there be such fixed points? The arguments above depend on a well-known fixed point theorem (due to Schauder) that guarantees the existence of a fixed point for compact, convex state spaces. We do not know what subsequent extensions of this result imply regarding fixed points for a wider variety of systems, or whether there are other general results along these lines. (See Kutach 2003 for more on this issue.)
A further interesting question is whether this line of argument is sufficient to resolve Consistency (see also Dowe 2007). When they apply, these results establish the existence of a solution, such as the shade of uniform gray in the first example. But physicists routinely demand more than merely the existence of a solution, namely that solutions to the equations are stable—such that “small” changes of the initial state lead to “small” changes of the resulting trajectory. (Clarifying the two senses of “small” in this statement requires further work, specifying the relevant topology.) Stability in this sense underwrites the possibility of applying equations to real systems given our inability to fix initial states with indefinite precision. (See Fletcher 2020 for further discussion.) The fixed point theorems guarantee that for an initial state \(S_1\) there is a solution, but this solution may not be “close” to the solution for a nearby initial state, \(S'\). We are not aware of any proofs that the solutions guaranteed to exist by the fixed point theorems are also stable in this sense.
Time travel has recently been discussed quite extensively in the context of general relativity. General relativity places few constraints on the global structure of space and time. This flexibility leads to a possibility first described in print by Hermann Weyl:
Every world-point is the origin of the double-cone of the active future and the passive past [i.e., the two lobes of the light cone]. Whereas in the special theory of relativity these two portions are separated by an intervening region, it is certainly possible in the present case [i.e., general relativity] for the cone of the active future to overlap with that of the passive past; so that, in principle, it is possible to experience events now that will in part be an effect of my future resolves and actions. Moreover, it is not impossible for a world-line (in particular, that of my body), although it has a timelike direction at every point, to return to the neighborhood of a point which it has already once passed through. (Weyl 1918/1920 [1952: 274])
A time-like curve is simply a space-time trajectory such that the speed of light is never equaled or exceeded along this trajectory. Time-like curves represent possible trajectories of ordinary objects. In general relativity a curve that is everywhere timelike locally can nonetheless loop back on itself, forming a CTC. Weyl makes the point vividly in terms of the light cones: along such a curve, the future lobe of the light cone (the “active future”) intersects the past lobe of the light cone (the “passive past”). Traveling along such a curve one would never exceed the speed of light, and yet after a certain amount of (proper) time one would return to a point in space-time that one previously visited. Or, by staying close to such a CTC, one could come arbitrarily close to a point in space-time that one previously visited. General relativity, in a straightforward sense, allows time travel: there appear to be many space-times compatible with the fundamental equations of general relativity in which there are CTC’s. Space-time, for instance, could have a Minkowski metric everywhere, and yet have CTC’s everywhere by having the temporal dimension (topologically) rolled up as a circle. Or, one can have wormhole connections between different parts of space-time which allow one to enter “mouth \(A\)” of such a wormhole connection, travel through the wormhole, exit the wormhole at “mouth \(B\)” and re-enter “mouth \(A\)” again. CTCs can even arise when the spacetime is topologically \(\mathbb{R}^4\), due to the “tilting” of light cones produced by rotating matter (as in Gödel 1949’s spacetime).
General relativity thus appears to provide ample opportunity for time travel. Note that just because there are CTC’s in a space-time, this does not mean that one can get from any point in the space-time to any other point by following some future directed timelike curve—there may be insurmountable practical obstacles. In Gödel’s spacetime, it is the case that there are CTCs passing through every point in the spacetime. Yet these CTCs are not geodesics, so traversing them requires acceleration. Calculations of the minimal fuel required to travel along the appropriate curve should discourage any would-be time travelers (Malament 1984, 1985; Manchak 2011). But more generally CTCs may be confined to smaller regions; some parts of space-time can have CTC’s while other parts do not. Let us call the part of a space-time that has CTC’s the “time travel region” of that space-time, while calling the rest of that space-time the “normal region”. More precisely, the “time travel region” consists of all the space-time points \(p\) such that there exists a (non-zero length) timelike curve that starts at \(p\) and returns to \(p\). Now let us turn to examining space-times with CTC’s a bit more closely for potential problems.
In order to get a feeling for the sorts of implications that closed timelike curves can have, it may be useful to consider two simple models. In space-times with closed timelike curves the traditional initial value problem cannot be framed in the usual way. For it presupposes the existence of Cauchy surfaces, and if there are CTCs then no Cauchy surface exists. (A Cauchy surface is a spacelike surface such that every inextendable timelike curve crosses it exactly once. One normally specifies initial conditions by giving the conditions on such a surface.) Nonetheless, if the topological complexities of the manifold are appropriately localized, we can come quite close. Let us call an edgeless spacelike surface \(S\) a quasi-Cauchy surface if it divides the rest of the manifold into two parts such that
- every point in the manifold can be connected by a timelike curve to \(S\), and
- any timelike curve which connects a point in one region to a point in the other region intersects \(S\) exactly once.
It is obvious that a quasi-Cauchy surface must entirely inhabit the normal region of the space-time; if any point \(p\) of \(S\) is in the time travel region, then any timelike curve which intersects \(p\) can be extended to a timelike curve which intersects \(S\) near \(p\) again. In extreme cases of time travel, a model may have no normal region at all (e.g., Minkowski space-time rolled up like a cylinder in a time-like direction), in which case our usual notions of temporal precedence will not apply. But temporal anomalies like wormholes (and time machines) can be sufficiently localized to permit the existence of quasi-Cauchy surfaces.
Given a timelike orientation, a quasi-Cauchy surface unproblematically divides the manifold into its past (i.e., all points that can be reached by past-directed timelike curves from \(S)\) and its future (ditto mutatis mutandis ). If the whole past of \(S\) is in the normal region of the manifold, then \(S\) is a partial Cauchy surface : every inextendable timelike curve which exists to the past of \(S\) intersects \(S\) exactly once, but (if there is time travel in the future) not every inextendable timelike curve which exists to the future of \(S\) intersects \(S\). Now we can ask a particularly clear question: consider a manifold which contains a time travel region, but also has a partial Cauchy surface \(S\), such that all of the temporal funny business is to the future of \(S\). If all you could see were \(S\) and its past, you would not know that the space-time had any time travel at all. The question is: are there any constraints on the sort of data which can be put on \(S\) and continued to a global solution of the dynamics which are different from the constraints (if any) on the data which can be put on a Cauchy surface in a simply connected manifold and continued to a global solution? If there is time travel to our future, might we we able to tell this now, because of some implied oddity in the arrangement of present things?
It is not at all surprising that there might be constraints on the data which can be put on a locally space-like surface which passes through the time travel region: after all, we never think we can freely specify what happens on a space-like surface and on another such surface to its future, but in this case the surface at issue lies to its own future. But if there were particular constraints for data on a partial Cauchy surface then we would apparently need to have to rule out some sorts of otherwise acceptable states on \(S\) if there is to be time travel to the future of \(S\). We then might be able to establish that there will be no time travel in the future by simple inspection of the present state of the universe. As we will see, there is reason to suspect that such constraints on the partial Cauchy surface are non-generic. But we are getting ahead of ourselves: first let’s consider the effect of time travel on a very simple dynamics.
The simplest possible example is the Newtonian theory of perfectly elastic collisions among equally massive particles in one spatial dimension. The space-time is two-dimensional, so we can represent it initially as the Euclidean plane, and the dynamics is completely specified by two conditions. When particles are traveling freely, their world lines are straight lines in the space-time, and when two particles collide, they exchange momenta, so the collision looks like an “\(X\)” in space-time, with each particle changing its momentum at the impact. [ 2 ] The dynamics is purely local, in that one can check that a set of world-lines constitutes a model of the dynamics by checking that the dynamics is obeyed in every arbitrarily small region. It is also trivial to generate solutions from arbitrary initial data if there are no CTCs: given the initial positions and momenta of a set of particles, one simply draws a straight line from each particle in the appropriate direction and continues it indefinitely. Once all the lines are drawn, the worldline of each particle can be traced from collision to collision. The boundary value problem for this dynamics is obviously well-posed: any set of data at an instant yields a unique global solution, constructed by the method sketched above.
What happens if we change the topology of the space-time by hand to produce CTCs? The simplest way to do this is depicted in figure 3 : we cut and paste the space-time so it is no longer simply connected by identifying the line \(L-\) with the line \(L+\). Particles “going in” to \(L+\) from below “emerge” from \(L-\) , and particles “going in” to \(L-\) from below “emerge” from \(L+\).
Figure 3: Inserting CTCs by Cut and Paste. [An extended description of figure 3 is in the supplement.]
How is the boundary-value problem changed by this alteration in the space-time? Before the cut and paste, we can put arbitrary data on the simultaneity slice \(S\) and continue it to a unique solution. After the change in topology, \(S\) is no longer a Cauchy surface, since a CTC will never intersect it, but it is a partial Cauchy surface. So we can ask two questions. First, can arbitrary data on \(S\) always be continued to a global solution? Second, is that solution unique? If the answer to the first question is \(no\), then we have a backward-temporal constraint: the existence of the region with CTCs places constraints on what can happen on \(S\) even though that region lies completely to the future of \(S\). If the answer to the second question is \(no\), then we have an odd sort of indeterminism, analogous to the unwritten book: the complete physical state on \(S\) does not determine the physical state in the future, even though the local dynamics is perfectly deterministic and even though there is no other past edge to the space-time region in \(S\)’s future (i.e., there is nowhere else for boundary values to come from which could influence the state of the region).
In this case the answer to the first question is yes and to the second is no : there are no constraints on the data which can be put on \(S\), but those data are always consistent with an infinitude of different global solutions. The easy way to see that there always is a solution is to construct the minimal solution in the following way. Start drawing straight lines from \(S\) as required by the initial data. If a line hits \(L-\) from the bottom, just continue it coming out of the top of \(L+\) in the appropriate place, and if a line hits \(L+\) from the bottom, continue it emerging from \(L-\) at the appropriate place. Figure 4 represents the minimal solution for a single particle which enters the time-travel region from the left:
Figure 4: The Minimal Solution. [An extended description of figure 4 is in the supplement.]
The particle “travels back in time” three times. It is obvious that this minimal solution is a global solution, since the particle always travels inertially.
But the same initial state on \(S\) is also consistent with other global solutions. The new requirement imposed by the topology is just that the data going into \(L+\) from the bottom match the data coming out of \(L-\) from the top, and the data going into \(L-\) from the bottom match the data coming out of \(L+\) from the top. So we can add any number of vertical lines connecting \(L-\) and \(L+\) to a solution and still have a solution. For example, adding a few such lines to the minimal solution yields:
Figure 5: A Non-Minimal Solution. [An extended description of figure 5 is in the supplement.]
The particle now collides with itself twice: first before it reaches \(L+\) for the first time, and again shortly before it exits the CTC region. From the particle’s point of view, it is traveling to the right at a constant speed until it hits an older version of itself and comes to rest. It remains at rest until it is hit from the right by a younger version of itself, and then continues moving off, and the same process repeats later. It is clear that this is a global model of the dynamics, and that any number of distinct models could be generating by varying the number and placement of vertical lines.
Knowing the data on \(S\), then, gives us only incomplete information about how things will go for the particle. We know that the particle will enter the CTC region, and will reach \(L+\), we know that it will be the only particle in the universe, we know exactly where and with what speed it will exit the CTC region. But we cannot determine how many collisions the particle will undergo (if any), nor how long (in proper time) it will stay in the CTC region. If the particle were a clock, we could not predict what time it would indicate when exiting the region. Furthermore, the dynamics gives us no handle on what to think of the various possibilities: there are no probabilities assigned to the various distinct possible outcomes.
Changing the topology has changed the mathematics of the situation in two ways, which tend to pull in opposite directions. On the one hand, \(S\) is no longer a Cauchy surface, so it is perhaps not surprising that data on \(S\) do not suffice to fix a unique global solution. But on the other hand, there is an added constraint: data “coming out” of \(L-\) must exactly match data “going in” to \(L+\), even though what comes out of \(L-\) helps to determine what goes into \(L+\). This added consistency constraint tends to cut down on solutions, although in this case the additional constraint is more than outweighed by the freedom to consider various sorts of data on \({L+}/{L-}\).
The fact that the extra freedom outweighs the extra constraint also points up one unexpected way that the supposed paradoxes of time travel may be overcome. Let’s try to set up a paradoxical situation using the little closed time loop above. If we send a single particle into the loop from the left and do nothing else, we know exactly where it will exit the right side of the time travel region. Now suppose we station someone at the other side of the region with the following charge: if the particle should come out on the right side, the person is to do something to prevent the particle from going in on the left in the first place. In fact, this is quite easy to do: if we send a particle in from the right, it seems that it can exit on the left and deflect the incoming left-hand particle.
Carrying on our reflection in this way, we further realize that if the particle comes out on the right, we might as well send it back in order to deflect itself from entering in the first place. So all we really need to do is the following: set up a perfectly reflecting particle mirror on the right-hand side of the time travel region, and launch the particle from the left so that— if nothing interferes with it —it will just barely hit \(L+\). Our paradox is now apparently complete. If, on the one hand, nothing interferes with the particle it will enter the time-travel region on the left, exit on the right, be reflected from the mirror, re-enter from the right, and come out on the left to prevent itself from ever entering. So if it enters, it gets deflected and never enters. On the other hand, if it never enters then nothing goes in on the left, so nothing comes out on the right, so nothing is reflected back, and there is nothing to deflect it from entering. So if it doesn’t enter, then there is nothing to deflect it and it enters. If it enters, then it is deflected and doesn’t enter; if it doesn’t enter then there is nothing to deflect it and it enters: paradox complete.
But at least one solution to the supposed paradox is easy to construct: just follow the recipe for constructing the minimal solution, continuing the initial trajectory of the particle (reflecting it the mirror in the obvious way) and then read of the number and trajectories of the particles from the resulting diagram. We get the result of figure 6 :
Figure 6: Resolving the “Paradox”. [An extended description of figure 6 is in the supplement.]
As we can see, the particle approaching from the left never reaches \(L+\): it is deflected first by a particle which emerges from \(L-\). But it is not deflected by itself , as the paradox suggests, it is deflected by another particle. Indeed, there are now four particles in the diagram: the original particle and three particles which are confined to closed time-like curves. It is not the leftmost particle which is reflected by the mirror, nor even the particle which deflects the leftmost particle; it is another particle altogether.
The paradox gets it traction from an incorrect presupposition. If there is only one particle in the world at \(S\) then there is only one particle which could participate in an interaction in the time travel region: the single particle would have to interact with its earlier (or later) self. But there is no telling what might come out of \(L-\): the only requirement is that whatever comes out must match what goes in at \(L+\). So if you go to the trouble of constructing a working time machine, you should be prepared for a different kind of disappointment when you attempt to go back and kill yourself: you may be prevented from entering the machine in the first place by some completely unpredictable entity which emerges from it. And once again a peculiar sort of indeterminism appears: if there are many self-consistent things which could prevent you from entering, there is no telling which is even likely to materialize. This is just like the case of the unwritten book: the book is never written, so nothing determines what fills its pages.
So when the freedom to put data on \(L-\) outweighs the constraint that the same data go into \(L+\), instead of paradox we get an embarrassment of riches: many solution consistent with the data on \(S\), or many possible books. To see a case where the constraint “outweighs” the freedom, we need to construct a very particular, and frankly artificial, dynamics and topology. Consider the space of all linear dynamics for a scalar field on a lattice. (The lattice can be though of as a simple discrete space-time.) We will depict the space-time lattice as a directed graph. There is to be a scalar field defined at every node of the graph, whose value at a given node depends linearly on the values of the field at nodes which have arrows which lead to it. Each edge of the graph can be assigned a weighting factor which determines how much the field at the input node contributes to the field at the output node. If we name the nodes by the letters a , b , c , etc., and the edges by their endpoints in the obvious way, then we can label the weighting factors by the edges they are associated with in an equally obvious way.
Suppose that the graph of the space-time lattice is acyclic , as in figure 7 . (A graph is Acyclic if one can not travel in the direction of the arrows and go in a loop.)
Figure 7: An Acyclic Lattice. [An extended description of figure 7 is in the supplement.]
It is easy to regard a set of nodes as the analog of a Cauchy surface, e.g., the set \(\{a, b, c\}\), and it is obvious if arbitrary data are put on those nodes the data will generate a unique solution in the future. [ 3 ] If the value of the field at node \(a\) is 3 and at node \(b\) is 7, then its value at node \(d\) will be \(3W_{ad}\) and its value at node \(e\) will be \(3W_{ae} + 7W_{be}\). By varying the weighting factors we can adjust the dynamics, but in an acyclic graph the future evolution of the field will always be unique.
Let us now again artificially alter the topology of the lattice to admit CTCs, so that the graph now is cyclic. One of the simplest such graphs is depicted in figure 8 : there are now paths which lead from \(z\) back to itself, e.g., \(z\) to \(y\) to \(z\).
Figure 8: Time Travel on a Lattice. [An extended description of figure 8 is in the supplement.]
Can we now put arbitrary data on \(v\) and \(w\), and continue that data to a global solution? Will the solution be unique?
In the generic case, there will be a solution and the solution will be unique. The equations for the value of the field at \(x, y\), and \(z\) are:
Solving these equations for \(z\) yields
which gives a unique value for \(z\) in the generic case. But looking at the space of all possible dynamics for this lattice (i.e., the space of all possible weighting factors), we find a singularity in the case where \(1-W_{zx}W_{xz} - W_{zy}W_{yz} = 0\). If we choose weighting factors in just this way, then arbitrary data at \(v\) and \(w\) cannot be continued to a global solution. Indeed, if the scalar field is everywhere non-negative, then this particular choice of dynamics puts ironclad constraints on the value of the field at \(v\) and \(w\): the field there must be zero (assuming \(W_{vx}\) and \(W_{wy}\) to be non-zero), and similarly all nodes in their past must have field value zero. If the field can take negative values, then the values at \(v\) and \(w\) must be so chosen that \(vW_{vx}W_{xz} = -wW_{wy}W_{yz}\). In either case, the field values at \(v\) and \(w\) are severely constrained by the existence of the CTC region even though these nodes lie completely to the past of that region. It is this sort of constraint which we find to be unlike anything which appears in standard physics.
Our toy models suggest three things. The first is that it may be impossible to prove in complete generality that arbitrary data on a partial Cauchy surface can always be continued to a global solution: our artificial case provides an example where it cannot. The second is that such odd constraints are not likely to be generic: we had to delicately fine-tune the dynamics to get a problem. The third is that the opposite problem, namely data on a partial Cauchy surface being consistent with many different global solutions, is likely to be generic: we did not have to do any fine-tuning to get this result.
This third point leads to a peculiar sort of indeterminism, illustrated by the case of the unwritten book: the entire state on \(S\) does not determine what will happen in the future even though the local dynamics is deterministic and there are no other “edges” to space-time from which data could influence the result. What happens in the time travel region is constrained but not determined by what happens on \(S\), and the dynamics does not even supply any probabilities for the various possibilities. The example of the photographic negative discussed in section 2, then, seems likely to be unusual, for in that case there is a unique fixed point for the dynamics, and the set-up plus the dynamical laws determine the outcome. In the generic case one would rather expect multiple fixed points, with no room for anything to influence, even probabilistically, which would be realized. (See the supplement on
Remarks and Limitations on the Toy Models .
It is ironic that time travel should lead generically not to contradictions or to constraints (in the normal region) but to underdetermination of what happens in the time travel region by what happens everywhere else (an underdetermination tied neither to a probabilistic dynamics nor to a free edge to space-time). The traditional objection to time travel is that it leads to contradictions: there is no consistent way to complete an arbitrarily constructed story about how the time traveler intends to act. Instead, though, it appears that the more significant problem is underdetermination: the story can be consistently completed in many different ways.
Echeverria, Klinkhammer, and Thorne (1991) considered the case of 3-dimensional single hard spherical ball that can go through a single time travel wormhole so as to collide with its younger self.
Figure 9 [An extended description of figure 9 is in the supplement.]
The threat of paradox in this case arises in the following form. Consider the initial trajectory of a ball as it approaches the time travel region. For some initial trajectories, the ball does not undergo a collision before reaching mouth 1, but upon exiting mouth 2 it will collide with its earlier self. This leads to a contradiction if the collision is strong enough to knock the ball off its trajectory and deflect it from entering mouth 1. Of course, the Wheeler-Feynman strategy is to look for a “glancing blow” solution: a collision which will produce exactly the (small) deviation in trajectory of the earlier ball that produces exactly that collision. Are there always such solutions? [ 4 ]
Echeverria, Klinkhammer & Thorne found a large class of initial trajectories that have consistent “glancing blow” continuations, and found none that do not (but their search was not completely general). They did not produce a rigorous proof that every initial trajectory has a consistent continuation, but suggested that it is very plausible that every initial trajectory has a consistent continuation. That is to say, they have made it very plausible that, in the billiard ball wormhole case, the time travel structure of such a wormhole space-time does not result in constraints on states on spacelike surfaces in the non-time travel region.
In fact, as one might expect from our discussion in the previous section, they found the opposite problem from that of inconsistency: they found underdetermination. For a large class of initial trajectories there are multiple different consistent “glancing blow” continuations of that trajectory (many of which involve multiple wormhole traversals). For example, if one initially has a ball that is traveling on a trajectory aimed straight between the two mouths, then one obvious solution is that the ball passes between the two mouths and never time travels. But another solution is that the younger ball gets knocked into mouth 1 exactly so as to come out of mouth 2 and produce that collision. Echeverria et al. do not note the possibility (which we pointed out in the previous section) of the existence of additional balls in the time travel region. We conjecture (but have no proof) that for every initial trajectory of \(A\) there are some, and generically many, multiple-ball continuations.
Friedman, Morris, et al. (1990) examined the case of source-free non-self-interacting scalar fields traveling through such a time travel wormhole and found that no constraints on initial conditions in the non-time travel region are imposed by the existence of such time travel wormholes. In general there appear to be no known counter examples to the claim that in “somewhat realistic” time-travel space-times with a partial Cauchy surface there are no constraints imposed on the state on such a partial Cauchy surface by the existence of CTC’s. (See, e.g., Friedman & Morris 1991; Thorne 1994; Earman 1995; Earman, Smeenk, & Wüthrich 2009; and Dowe 2007.)
How about the issue of constraints in the time travel region \(T\)? Prima facie , constraints in such a region would not appear to be surprising. But one might still expect that there should be no constraints on states on a spacelike surface, provided one keeps the surface “small enough”. In the physics literature the following question has been asked: for any point \(p\) in \(T\), and any space-like surface \(S\) that includes \(p\) is there a neighborhood \(E\) of \(p\) in \(S\) such that any solution on \(E\) can be extended to a solution on the whole space-time? With respect to this question, there are some simple models in which one has this kind of extendability of local solutions to global ones, and some simple models in which one does not have such extendability, with no clear general pattern. The technical mathematical problems are amplified by the more conceptual problem of what it might mean to say that one could create a situation which forces the creation of closed timelike curves. (See, e.g., Yurtsever 1990; Friedman, Morris, et al. 1990; Novikov 1992; Earman 1995; and Earman, Smeenk, & Wüthrich 2009). What are we to think of all of this?
The toy models above all treat billiard balls, fields, and other objects propagating through a background spacetime with CTCs. Even if we can show that a consistent solution exists, there is a further question: what kind of matter and dynamics could generate CTCs to begin with? There are various solutions of Einstein’s equations with CTCs, but how do these exotic spacetimes relate to the models actually used in describing the world? In other words, what positive reasons might we have to take CTCs seriously as a feature of the actual universe, rather than an exotic possibility of primarily mathematical interest?
We should distinguish two different kinds of “possibility” that we might have in mind in posing such questions (following Stein 1970). First, we can consider a solution as a candidate cosmological model, describing the (large-scale gravitational degrees of freedom of the) entire universe. The case for ruling out spacetimes with CTCs as potential cosmological models strikes us as, surprisingly, fairly weak. Physicists used to simply rule out solutions with CTCs as unreasonable by fiat, due to the threat of paradoxes, which we have dismantled above. But it is also challenging to make an observational case. Observations tell us very little about global features, such as the existence of CTCs, because signals can only reach an observer from a limited region of spacetime, called the past light cone. Our past light cone—and indeed the collection of all the past light cones for possible observers in a given spacetime—can be embedded in spacetimes with quite different global features (Malament 1977, Manchak 2009). This undercuts the possibility of using observations to constrain global topology, including (among other things) ruling out the existence of CTCs.
Yet the case in favor of taking cosmological models with CTCs seriously is also not particularly strong. Some solutions used to describe black holes, which are clearly relevant in a variety of astrophysical contexts, include CTCs. But the question of whether the CTCs themselves play an essential representational role is subtle: the CTCs arise in the maximal extensions of these solutions, and can plausibly be regarded as extraneous to successful applications. Furthermore, many of the known solutions with CTCs have symmetries, raising the possibility that CTCs are not a stable or robust feature. Slight departures from symmetry may lead to a solution without CTCs, suggesting that the CTCs may be an artifact of an idealized model.
The second sense of possibility regards whether “reasonable” initial conditions can be shown to lead to, or not to lead to, the formation of CTCs. As with the toy models above, suppose that we have a partial Cauchy surface \(S\), such that all the temporal funny business lies to the future. Rather than simply assuming that there is a region with CTCs to the future, we can ask instead whether it is possible to create CTCs by manipulating matter in the initial, well-behaved region—that is, whether it is possible to build a time machine. Several physicists have pursued “chronology protection theorems” aiming to show that the dynamics of general relativity (or some other aspects of physics) rules this out, and to clarify why this is the case. The proof of such a theorem would justify neglecting solutions with CTCs as a source of insight into the nature of time in the actual world. But as of yet there are several partial results that do not fully settle the question. One further intriguing possibility is that even if general relativity by itself does protect chronology, it may not be possible to formulate a sensible theory describing matter and fields in solutions with CTCs. (See SEP entry on Time Machines; Smeenk and Wüthrich 2011 for more.)
There is a different question regarding the limitations of these toy models. The toy models and related examples show that there are consistent solutions for simple systems in the presence of CTCs. As usual we have made the analysis tractable by building toy models, selecting only a few dynamical degrees of freedom and tracking their evolution. But there is a large gap between the systems we have described and the time travel stories they evoke, with Kurt traveling along a CTC with murderous intentions. In particular, many features of the manifest image of time are tied to the thermodynamical properties of macroscopic systems. Rovelli (unpublished) considers a extremely simple system to illustrate the problem: can a clock move along a CTC? A clock consists of something in periodic motion, such as a pendulum bob, and something that counts the oscillations, such as an escapement mechanism. The escapement mechanism cannot work without friction; this requires dissipation and increasing entropy. For a clock that counts oscillations as it moves along a time-like trajectory, the entropy must be a monotonically increasing function. But that is obviously incompatible with the clock returning to precisely the same state at some future time as it completes a loop. The point generalizes, obviously, to imply that anything like a human, with memory and agency, cannot move along a CTC.
Since it is not obvious that one can rid oneself of all constraints in realistic models, let us examine the argument that time travel is implausible, and we should think it unlikely to exist in our world, in so far as it implies such constraints. The argument goes something like the following. In order to satisfy such constraints one needs some pre-established divine harmony between the global (time travel) structure of space-time and the distribution of particles and fields on space-like surfaces in it. But it is not plausible that the actual world, or any world even remotely like ours, is constructed with divine harmony as part of the plan. In fact, one might argue, we have empirical evidence that conditions in any spatial region can vary quite arbitrarily. So we have evidence that such constraints, whatever they are, do not in fact exist in our world. So we have evidence that there are no closed time-like lines in our world or one remotely like it. We will now examine this argument in more detail by presenting four possible responses, with counterresponses, to this argument.
Response 1. There is nothing implausible or new about such constraints. For instance, if the universe is spatially closed, there has to be enough matter to produce the needed curvature, and this puts constraints on the matter distribution on a space-like hypersurface. Thus global space-time structure can quite unproblematically constrain matter distributions on space-like hypersurfaces in it. Moreover we have no realistic idea what these constraints look like, so we hardly can be said to have evidence that they do not obtain.
Counterresponse 1. Of course there are constraining relations between the global structure of space-time and the matter in it. The Einstein equations relate curvature of the manifold to the matter distribution in it. But what is so strange and implausible about the constraints imposed by the existence of closed time-like curves is that these constraints in essence have nothing to do with the Einstein equations. When investigating such constraints one typically treats the particles and/or field in question as test particles and/or fields in a given space-time, i.e., they are assumed not to affect the metric of space-time in any way. In typical space-times without closed time-like curves this means that one has, in essence, complete freedom of matter distribution on a space-like hypersurface. (See response 2 for some more discussion of this issue). The constraints imposed by the possibility of time travel have a quite different origin and are implausible. In the ordinary case there is a causal interaction between matter and space-time that results in relations between global structure of space-time and the matter distribution in it. In the time travel case there is no such causal story to be told: there simply has to be some pre-established harmony between the global space-time structure and the matter distribution on some space-like surfaces. This is implausible.
Response 2. Constraints upon matter distributions are nothing new. For instance, Maxwell’s equations constrain electric fields \(\boldsymbol{E}\) on an initial surface to be related to the (simultaneous) charge density distribution \(\varrho\) by the equation \(\varrho = \text{div}(\boldsymbol{E})\). (If we assume that the \(E\) field is generated solely by the charge distribution, this conditions amounts to requiring that the \(E\) field at any point in space simply be the one generated by the charge distribution according to Coulomb’s inverse square law of electrostatics.) This is not implausible divine harmony. Such constraints can hold as a matter of physical law. Moreover, if we had inferred from the apparent free variation of conditions on spatial regions that there could be no such constraints we would have mistakenly inferred that \(\varrho = \text{div}(\boldsymbol{E})\) could not be a law of nature.
Counterresponse 2. The constraints imposed by the existence of closed time-like lines are of quite a different character from the constraint imposed by \(\varrho = \text{div}(\boldsymbol{E})\). The constraints imposed by \(\varrho = \text{div}(\boldsymbol{E})\) on the state on a space-like hypersurface are:
- local constraints (i.e., to check whether the constraint holds in a region you just need to see whether it holds at each point in the region),
- quite independent of the global space-time structure,
- quite independent of how the space-like surface in question is embedded in a given space-time, and
- very simply and generally stateable.
On the other hand, the consistency constraints imposed by the existence of closed time-like curves (i) are not local, (ii) are dependent on the global structure of space-time, (iii) depend on the location of the space-like surface in question in a given space-time, and (iv) appear not to be simply stateable other than as the demand that the state on that space-like surface embedded in such and such a way in a given space-time, do not lead to inconsistency. On some views of laws (e.g., David Lewis’ view) this plausibly implies that such constraints, even if they hold, could not possibly be laws. But even if one does not accept such a view of laws, one could claim that the bizarre features of such constraints imply that it is implausible that such constraints hold in our world or in any world remotely like ours.
Response 3. It would be strange if there are constraints in the non-time travel region. It is not strange if there are constraints in the time travel region. They should be explained in terms of the strange, self-interactive, character of time travel regions. In this region there are time-like trajectories from points to themselves. Thus the state at such a point, in such a region, will, in a sense, interact with itself. It is a well-known fact that systems that interact with themselves will develop into an equilibrium state, if there is such an equilibrium state, or else will develop towards some singularity. Normally, of course, self-interaction isn’t true instantaneous self-interaction, but consists of a feed-back mechanism that takes time. But in time travel regions something like true instantaneous self-interaction occurs. This explains why constraints on states occur in such time travel regions: the states “ ab initio ” have to be “equilibrium states”. Indeed in a way this also provides some picture of why indeterminism occurs in time travel regions: at the onset of self-interaction states can fork into different equi-possible equilibrium states.
Counterresponse 3. This is explanation by woolly analogy. It all goes to show that time travel leads to such bizarre consequences that it is unlikely that it occurs in a world remotely like ours.
Response 4. All of the previous discussion completely misses the point. So far we have been taking the space-time structure as given, and asked the question whether a given time travel space-time structure imposes constraints on states on (parts of) space-like surfaces. However, space-time and matter interact. Suppose that one is in a space-time with closed time-like lines, such that certain counterfactual distributions of matter on some neighborhood of a point \(p\) are ruled out if one holds that space-time structure fixed. One might then ask
Why does the actual state near \(p\) in fact satisfy these constraints? By what divine luck or plan is this local state compatible with the global space-time structure? What if conditions near \(p\) had been slightly different?
And one might take it that the lack of normal answers to these questions indicates that it is very implausible that our world, or any remotely like it, is such a time travel universe. However the proper response to these question is the following. There are no constraints in any significant sense. If they hold they hold as a matter of accidental fact, not of law. There is no more explanation of them possible than there is of any contingent fact. Had conditions in a neighborhood of \(p\) been otherwise, the global structure of space-time would have been different. So what? The only question relevant to the issue of constraints is whether an arbitrary state on an arbitrary spatial surface \(S\) can always be embedded into a space-time such that that state on \(S\) consistently extends to a solution on the entire space-time.
But we know the answer to that question. A well-known theorem in general relativity says the following: any initial data set on a three dimensional manifold \(S\) with positive definite metric has a unique embedding into a maximal space-time in which \(S\) is a Cauchy surface (see, e.g., Geroch & Horowitz 1979: 284 for more detail), i.e., there is a unique largest space-time which has \(S\) as a Cauchy surface and contains a consistent evolution of the initial value data on \(S\). Now since \(S\) is a Cauchy surface this space-time does not have closed time like curves. But it may have extensions (in which \(S\) is not a Cauchy surface) which include closed timelike curves, indeed it may be that any maximal extension of it would include closed timelike curves. (This appears to be the case for extensions of states on certain surfaces of Taub-NUT space-times. See Earman, Smeenk, & Wüthrich 2009). But these extensions, of course, will be consistent. So properly speaking, there are no constraints on states on space-like surfaces. Nonetheless the space-time in which these are embedded may or may not include closed time-like curves.
Counterresponse 4. This, in essence, is the stonewalling answer which we indicated in section 1. However, whether or not you call the constraints imposed by a given space-time on distributions of matter on certain space-like surfaces “genuine constraints”, whether or not they can be considered lawlike, and whether or not they need to be explained, the existence of such constraints can still be used to argue that time travel worlds are so bizarre that it is implausible that our world or any world remotely like ours is a time travel world.
Suppose that one is in a time travel world. Suppose that given the global space-time structure of this world, there are constraints imposed upon, say, the state of motion of a ball on some space-like surface when it is treated as a test particle, i.e., when it is assumed that the ball does not affect the metric properties of the space-time it is in. (There is lots of other matter that, via the Einstein equation, corresponds exactly to the curvature that there is everywhere in this time travel worlds.) Now a real ball of course does have some effect on the metric of the space-time it is in. But let us consider a ball that is so small that its effect on the metric is negligible. Presumably it will still be the case that certain states of this ball on that space-like surface are not compatible with the global time travel structure of this universe.
This means that the actual distribution of matter on such a space-like surface can be extended into a space-time with closed time-like lines, but that certain counterfactual distributions of matter on this space-like surface can not be extended into the same space-time. But note that the changes made in the matter distribution (when going from the actual to the counterfactual distribution) do not in any non-negligible way affect the metric properties of the space-time. (Recall that the changes only effect test particles.) Thus the reason why the global time travel properties of the counterfactual space-time have to be significantly different from the actual space-time is not that there are problems with metric singularities or alterations in the metric that force significant global changes when we go to the counterfactual matter distribution. The reason that the counterfactual space-time has to be different is that in the counterfactual world the ball’s initial state of motion starting on the space-like surface, could not “meet up” in a consistent way with its earlier self (could not be consistently extended) if we were to let the global structure of the counterfactual space-time be the same as that of the actual space-time. Now, it is not bizarre or implausible that there is a counterfactual dependence of manifold structure, even of its topology, on matter distributions on spacelike surfaces. For instance, certain matter distributions may lead to singularities, others may not. We may indeed in some sense have causal power over the topology of the space-time we live in. But this power normally comes via the Einstein equations. But it is bizarre to think that there could be a counterfactual dependence of global space-time structure on the arrangement of certain tiny bits of matter on some space-like surface, where changes in that arrangement by assumption do not affect the metric anywhere in space-time in any significant way . It is implausible that we live in such a world, or that a world even remotely like ours is like that.
Let us illustrate this argument in a different way by assuming that wormhole time travel imposes constraints upon the states of people prior to such time travel, where the people have so little mass/energy that they have negligible effect, via the Einstein equation, on the local metric properties of space-time. Do you think it more plausible that we live in a world where wormhole time travel occurs but it only occurs when people’s states are such that these local states happen to combine with time travel in such a way that nobody ever succeeds in killing their younger self, or do you think it more plausible that we are not in a wormhole time travel world? [ 5 ]
An alternative approach to time travel (initiated by Deutsch 1991) abstracts away from the idealized toy models described above. [ 6 ] This computational approach considers instead the evolution of bits (simple physical systems with two discrete states) through a network of interactions, which can be represented by a circuit diagram with gates corresponding to the interactions. Motivated by the possibility of CTCs, Deutsch proposed adding a new kind of channel that connects the output of a given gate back to its input —in essence, a backwards-time step. More concretely, given a gate that takes \(n\) bits as input, we can imagine taking some number \(i \lt n\) of these bits through a channel that loops back and then do double-duty as inputs. Consistency requires that the state of these \(i\) bits is the same for output and input. (We will consider an illustration of this kind of system in the next section.) Working through examples of circuit diagrams with a CTC channel leads to similar treatments of Consistency and Underdetermination as the discussion above (see, e.g., Wallace 2012: § 10.6). But the approach offers two new insights (both originally due to Deutsch): the Easy Knowledge paradox, and a particularly clear extension to time travel in quantum mechanics.
A computer equipped with a CTC channel can exploit the need to find consistent evolution to solve remarkably hard problems. (This is quite different than the first idea that comes to mind to enhance computational power: namely to just devote more time to a computation, and then send the result back on the CTC to an earlier state.) The gate in a circuit incorporating a CTC implements a function from the input bits to the output bits, under the constraint that the output and input match the i bits going through the CTC channel. This requires, in effect, finding the fixed point of the relevant function. Given the generality of the model, there are few limits on the functions that could be implemented on the CTC circuit. Nature has to solve a hard computational problem just to ensure consistent evolution. This can then be extended to other complex computational problems—leading, more precisely, to solutions of NP -complete problems in polynomial time (see Aaronson 2013: Chapter 20 for an overview and further references). The limits imposed by computational complexity are an essential part of our epistemic situation, and computers with CTCs would radically change this.
We now turn to the application of the computational approach to the quantum physics of time travel (see Deutsch 1991; Deutsch & Lockwood 1994). By contrast with the earlier discussions of constraints in classical systems, they claim to show that time travel never imposes any constraints on the pre-time travel state of quantum systems. The essence of this account is as follows. [ 7 ]
A quantum system starts in state \(S_1\), interacts with its older self, after the interaction is in state \(S_2\), time travels while developing into state \(S_3\), then interacts with its younger self, and ends in state \(S_4\) (see figure 10 ).
Figure 10 [An extended description of figure 10 is in the supplement.]
Deutsch assumes that the set of possible states of this system are the mixed states, i.e., are represented by the density matrices over the Hilbert space of that system. Deutsch then shows that for any initial state \(S_1\), any unitary interaction between the older and younger self, and any unitary development during time travel, there is a consistent solution, i.e., there is at least one pair of states \(S_2\) and \(S_3\) such that when \(S_1\) interacts with \(S_3\) it will change to state \(S_2\) and \(S_2\) will then develop into \(S_3\). The states \(S_2, S_3\) and \(S_4\) will typically be not be pure states, i.e., will be non-trivial mixed states, even if \(S_1\) is pure. In order to understand how this leads to interpretational problems let us give an example. Consider a system that has a two dimensional Hilbert space with as a basis the states \(\vc{+}\) and \(\vc{-}\). Let us suppose that when state \(\vc{+}\) of the young system encounters state \(\vc{+}\) of the older system, they interact and the young system develops into state \(\vc{-}\) and the old system remains in state \(\vc{+}\). In obvious notation:
Similarly, suppose that:
Let us furthermore assume that there is no development of the state of the system during time travel, i.e., that \(\vc{+}_2\) develops into \(\vc{+}_3\), and that \(\vc{-}_2\) develops into \(\vc{-}_3\).
Now, if the only possible states of the system were \(\vc{+}\) and \(\vc{-}\) (i.e., if there were no superpositions or mixtures of these states), then there is a constraint on initial states: initial state \(\vc{+}_1\) is impossible. For if \(\vc{+}_1\) interacts with \(\vc{+}_3\) then it will develop into \(\vc{-}_2\), which, during time travel, will develop into \(\vc{-}_3\), which inconsistent with the assumed state \(\vc{+}_3\). Similarly if \(\vc{+}_1\) interacts with \(\vc{-}_3\) it will develop into \(\vc{+}_2\), which will then develop into \(\vc{+}_3\) which is also inconsistent. Thus the system can not start in state \(\vc{+}_1\).
But, says Deutsch, in quantum mechanics such a system can also be in any mixture of the states \(\vc{+}\) and \(\vc{-}\). Suppose that the older system, prior to the interaction, is in a state \(S_3\) which is an equal mixture of 50% \(\vc{+}_3\) and 50% \(\vc{-}_3\). Then the younger system during the interaction will develop into a mixture of 50% \(\vc{+}_2\) and 50% \(\vc{-}_2\), which will then develop into a mixture of 50% \(\vc{+}_3\) and 50% \(\vc{-}_3\), which is consistent! More generally Deutsch uses a fixed point theorem to show that no matter what the unitary development during interaction is, and no matter what the unitary development during time travel is, for any state \(S_1\) there is always a state \(S_3\) (which typically is not a pure state) which causes \(S_1\) to develop into a state \(S_2\) which develops into that state \(S_3\). Thus quantum mechanics comes to the rescue: it shows in all generality that no constraints on initial states are needed!
One might wonder why Deutsch appeals to mixed states: will superpositions of states \(\vc{+}\) and \(\vc{-}\) not suffice? Unfortunately such an idea does not work. Suppose again that the initial state is \(\vc{+}_1\). One might suggest that that if state \(S_3\) is
one will obtain a consistent development. For one might think that when initial state \(\vc{+}_1\) encounters the superposition
it will develop into superposition
and that this in turn will develop into
as desired. However this is not correct. For initial state \(\vc{+}_1\) when it encounters
will develop into the entangled state
In so far as one can speak of the state of the young system after this interaction, it is in the mixture of 50% \(\vc{+}_2\) and 50% \(\vc{-}_2\), not in the superposition
So Deutsch does need his recourse to mixed states.
This clarification of why Deutsch needs his mixtures does however indicate a serious worry about the simplifications that are part of Deutsch’s account. After the interaction the old and young system will (typically) be in an entangled state. Although for purposes of a measurement on one of the two systems one can say that this system is in a mixed state, one can not represent the full state of the two systems by specifying the mixed state of each separate part, as there are correlations between observables of the two systems that are not represented by these two mixed states, but are represented in the joint entangled state. But if there really is an entangled state of the old and young systems directly after the interaction, how is one to represent the subsequent development of this entangled state? Will the state of the younger system remain entangled with the state of the older system as the younger system time travels and the older system moves on into the future? On what space-like surfaces are we to imagine this total entangled state to be? At this point it becomes clear that there is no obvious and simple way to extend elementary non-relativistic quantum mechanics to space-times with closed time-like curves: we apparently need to characterize not just the entanglement between two systems, but entanglement relative to specific spacetime descriptions.
How does Deutsch avoid these complications? Deutsch assumes a mixed state \(S_3\) of the older system prior to the interaction with the younger system. He lets it interact with an arbitrary pure state \(S_1\) younger system. After this interaction there is an entangled state \(S'\) of the two systems. Deutsch computes the mixed state \(S_2\) of the younger system which is implied by this entangled state \(S'\). His demand for consistency then is just that this mixed state \(S_2\) develops into the mixed state \(S_3\). Now it is not at all clear that this is a legitimate way to simplify the problem of time travel in quantum mechanics. But even if we grant him this simplification there is a problem: how are we to understand these mixtures?
If we take an ignorance interpretation of mixtures we run into trouble. For suppose that we assume that in each individual case each older system is either in state \(\vc{+}_3\) or in state \(\vc{-}_3\) prior to the interaction. Then we regain our paradox. Deutsch instead recommends the following, many worlds, picture of mixtures. Suppose we start with state \(\vc{+}_1\) in all worlds. In some of the many worlds the older system will be in the \(\vc{+}_3\) state, let us call them A -worlds, and in some worlds, B -worlds, it will be in the \(\vc{-}_3\) state. Thus in A -worlds after interaction we will have state \(\vc{-}_2\) , and in B -worlds we will have state \(\vc{+}_2\). During time travel the \(\vc{-}_2\) state will remain the same, i.e., turn into state \(\vc{-}_3\), but the systems in question will travel from A -worlds to B -worlds. Similarly the \(\vc{+}\) \(_2\) states will travel from the B -worlds to the A -worlds, thus preserving consistency.
Now whatever one thinks of the merits of many worlds interpretations, and of this understanding of it applied to mixtures, in the end one does not obtain genuine time travel in Deutsch’s account. The systems in question travel from one time in one world to another time in another world, but no system travels to an earlier time in the same world. (This is so at least in the normal sense of the word “world”, the sense that one means when, for instance, one says “there was, and will be, only one Elvis Presley in this world.”) Thus, even if it were a reasonable view, it is not quite as interesting as it may have initially seemed. (See Wallace 2012 for a more sympathetic treatment, that explores several further implications of accepting time travel in conjunction with the many worlds interpretation.)
We close by acknowledging that Deutsch’s starting point—the claim that this computational model captures the essential features of quantum systems in a spacetime with CTCs—has been the subject of some debate. Several physicists have pursued a quite different treatment of evolution of quantum systems through CTC’s, based on considering the “post-selected” state (see Lloyd et al. 2011). Their motivations for implementing the consistency condition in terms of the post-selected state reflects a different stance towards quantum foundations. A different line of argument aims to determine whether Deutsch’s treatment holds as an appropriate limiting case of a more rigorous treatment, such as quantum field theory in curved spacetimes. For example, Verch (2020) establishes several results challenging the assumption that Deutsch’s treatment is tied to the presence of CTC’s, or that it is compatible with the entanglement structure of quantum fields.
What remains of the grandfather paradox in general relativistic time travel worlds is the fact that in some cases the states on edgeless spacelike surfaces are “overconstrained”, so that one has less than the usual freedom in specifying conditions on such a surface, given the time-travel structure, and in some cases such states are “underconstrained”, so that states on edgeless space-like surfaces do not determine what happens elsewhere in the way that they usually do, given the time travel structure. There can also be mixtures of those two types of cases. The extent to which states are overconstrained and/or underconstrained in realistic models is as yet unclear, though it would be very surprising if neither obtained. The extant literature has primarily focused on the problem of overconstraint, since that, often, either is regarded as a metaphysical obstacle to the possibility time travel, or as an epistemological obstacle to the plausibility of time travel in our world. While it is true that our world would be quite different from the way we normally think it is if states were overconstrained, underconstraint seems at least as bizarre as overconstraint. Nonetheless, neither directly rules out the possibility of time travel.
If time travel entailed contradictions then the issue would be settled. And indeed, most of the stories employing time travel in popular culture are logically incoherent: one cannot “change” the past to be different from what it was, since the past (like the present and the future) only occurs once. But if the only requirement demanded is logical coherence, then it seems all too easy. A clever author can devise a coherent time-travel scenario in which everything happens just once and in a consistent way. This is just too cheap: logical coherence is a very weak condition, and many things we take to be metaphysically impossible are logically coherent. For example, it involves no logical contradiction to suppose that water is not molecular, but if both chemistry and Kripke are right it is a metaphysical impossibility. We have been interested not in logical possibility but in physical possibility. But even so, our conditions have been relatively weak: we have asked only whether time-travel is consistent with the universal validity of certain fundamental physical laws and with the notion that the physical state on a surface prior to the time travel region be unconstrained. It is perfectly possible that the physical laws obey this condition, but still that time travel is not metaphysically possible because of the nature of time itself. Consider an analogy. Aristotle believed that water is homoiomerous and infinitely divisible: any bit of water could be subdivided, in principle, into smaller bits of water. Aristotle’s view contains no logical contradiction. It was certainly consistent with Aristotle’s conception of water that it be homoiomerous, so this was, for him, a conceptual possibility. But if chemistry is right, Aristotle was wrong both about what water is like and what is possible for it. It can’t be infinitely divided, even though no logical or conceptual analysis would reveal that.
Similarly, even if all of our consistency conditions can be met, it does not follow that time travel is physically possible, only that some specific physical considerations cannot rule it out. The only serious proof of the possibility of time travel would be a demonstration of its actuality. For if we agree that there is no actual time travel in our universe, the supposition that there might have been involves postulating a substantial difference from actuality, a difference unlike in kind from anything we could know if firsthand. It is unclear to us exactly what the content of possible would be if one were to either maintain or deny the possibility of time travel in these circumstances, unless one merely meant that the possibility is not ruled out by some delineated set of constraints. As the example of Aristotle’s theory of water shows, conceptual and logical “possibility” do not entail possibility in a full-blooded sense. What exactly such a full-blooded sense would be in case of time travel, and whether one could have reason to believe it to obtain, remain to us obscure.
- Aaronson, Scott, 2013, Quantum Computing since Democritus , Cambridge: Cambridge University Press. doi:10.1017/CBO9780511979309
- Arntzenius, Frank, 2006, “Time Travel: Double Your Fun”, Philosophy Compass , 1(6): 599–616. doi:10.1111/j.1747-9991.2006.00045.x
- Clarke, C.J.S., 1977, “Time in General Relativity” in Foundations of Space-Time Theory , Minnesota Studies in the Philosophy of Science , Vol VIII, Earman, J., Glymour, C., and Stachel, J. (eds), pp. 94–108. Minneapolis: University of Minnesota Press.
- Deutsch, David, 1991, “Quantum Mechanics near Closed Timelike Lines”, Physical Review D , 44(10): 3197–3217. doi:10.1103/PhysRevD.44.3197
- Deutsch, David and Michael Lockwood, 1994, “The Quantum Physics of Time Travel”, Scientific American , 270(3): 68–74. doi:10.1038/scientificamerican0394-68
- Dowe, Phil, 2007, “Constraints on Data in Worlds with Closed Timelike Curves”, Philosophy of Science , 74(5): 724–735. doi:10.1086/525617
- Earman, John, 1972, “Implications of Causal Propagation Outside the Null Cone”, Australasian Journal of Philosophy , 50(3): 222–237. doi:10.1080/00048407212341281
- Earman, John, 1995, Bangs, Crunches, Whimpers, and Shrieks: Singularities and Acausalities in Relativistic Spacetimes , New York: Oxford University Press.
- Earman, John, Christopher Smeenk, and Christian Wüthrich, 2009, “Do the Laws of Physics Forbid the Operation of Time Machines?”, Synthese , 169(1): 91–124. doi:10.1007/s11229-008-9338-2
- Echeverria, Fernando, Gunnar Klinkhammer, and Kip S. Thorne, 1991, “Billiard Balls in Wormhole Spacetimes with Closed Timelike Curves: Classical Theory”, Physical Review D , 44(4): 1077–1099. doi:10.1103/PhysRevD.44.1077
- Effingham, Nikk, 2020, Time Travel: Probability and Impossibility , Oxford: Oxford University Press. doi:10.1093/oso/9780198842507.001.0001
- Fletcher, Samuel C., 2020, “The Principle of Stability”, Philosopher’s Imprint , 20: article 3. [ Fletcher 2020 available online ]
- Friedman, John and Michael Morris, 1991, “The Cauchy Problem for the Scalar Wave Equation Is Well Defined on a Class of Spacetimes with Closed Timelike Curves”, Physical Review Letters , 66(4): 401–404. doi:10.1103/PhysRevLett.66.401
- Friedman, John, Michael S. Morris, Igor D. Novikov, Fernando Echeverria, Gunnar Klinkhammer, Kip S. Thorne, and Ulvi Yurtsever, 1990, “Cauchy Problem in Spacetimes with Closed Timelike Curves”, Physical Review D , 42(6): 1915–1930. doi:10.1103/PhysRevD.42.1915
- Geroch, Robert and Gary Horowitz, 1979, “Global Structures of Spacetimes”, in General Relativity: An Einstein Centenary Survey , Stephen Hawking and W. Israel (eds.), Cambridge/New York: Cambridge University Press, Chapter 5, pp. 212–293.
- Gödel, Kurt, 1949, “A Remark About the Relationship Between Relativity Theory and Idealistic Philosophy”, in Albert Einstein, Philosopher-Scientist , Paul Arthur Schilpp (ed.), Evanston, IL: Library of Living Philosophers, 557–562.
- Hocking, John G. and Gail S. Young, 1961, Topology , (Addison-Wesley Series in Mathematics), Reading, MA: Addison-Wesley.
- Horwich, Paul, 1987, “Time Travel”, in his Asymmetries in Time: Problems in the Philosophy of Science , , Cambridge, MA: MIT Press, 111–128.
- Kutach, Douglas N., 2003, “Time Travel and Consistency Constraints”, Philosophy of Science , 70(5): 1098–1113. doi:10.1086/377392
- Lewis, David, 1976, “The Paradoxes of Time Travel”, American Philosophical Quarterly , 13(2): 145–152.
- Lloyd, Seth, Lorenzo Maccone, Raul Garcia-Patron, Vittorio Giovannetti, and Yutaka Shikano, 2011, “Quantum Mechanics of Time Travel through Post-Selected Teleportation”, Physical Review D , 84(2): 025007. doi:10.1103/PhysRevD.84.025007
- Malament, David B., 1977, “Observationally Indistinguishable Spacetimes: Comments on Glymour’s Paper”, in Foundations of Space-Time Theories , John Earman, Clark N. Glymour, and John J. Stachel (eds.), (Minnesota Studies in the Philosophy of Science 8), Minneapolis, MN: University of Minnesota Press, 61–80.
- –––, 1984, “‘Time Travel’ in the Gödel Universe”, PSA: Proceedings of the Biennial Meeting of the Philosophy of Science Association , 1984(2): 91–100. doi:10.1086/psaprocbienmeetp.1984.2.192497
- –––, 1985, “Minimal Acceleration Requirements for ‘Time Travel’, in Gödel Space‐time”, Journal of Mathematical Physics , 26(4): 774–777. doi:10.1063/1.526566
- Manchak, John Byron, 2009, “Can We Know the Global Structure of Spacetime?”, Studies in History and Philosophy of Science Part B: Studies in History and Philosophy of Modern Physics , 40(1): 53–56. doi:10.1016/j.shpsb.2008.07.004
- –––, 2011, “On Efficient ‘Time Travel’ in Gödel Spacetime”, General Relativity and Gravitation , 43(1): 51–60. doi:10.1007/s10714-010-1068-3
- Maudlin, Tim, 1990, “Time-Travel and Topology”, PSA: Proceedings of the Biennial Meeting of the Philosophy of Science Association , 1990(1): 303–315. doi:10.1086/psaprocbienmeetp.1990.1.192712
- Novikov, I. D., 1992, “Time Machine and Self-Consistent Evolution in Problems with Self-Interaction”, Physical Review D , 45(6): 1989–1994. doi:10.1103/PhysRevD.45.1989
- Smeenk, Chris and Christian Wüthrich, 2011, “Time Travel and Time Machines”, in the Oxford Handbook on Time , Craig Callender (ed.), Oxford: Oxford University Press, 577–630..
- Stein, Howard, 1970, “On the Paradoxical Time-Structures of Gödel”, Philosophy of Science , 37(4): 589–601. doi:10.1086/288328
- Thorne, Kip S., 1994, Black Holes and Time Warps: Einstein’s Outrageous Legacy , (Commonwealth Fund Book Program), New York: W.W. Norton.
- Verch, Rainer, 2020, “The D-CTC Condition in Quantum Field Theory”, in Progress and Visions in Quantum Theory in View of Gravity , Felix Finster, Domenico Giulini, Johannes Kleiner, and Jürgen Tolksdorf (eds.), Cham: Springer International Publishing, 221–232. doi:10.1007/978-3-030-38941-3_9
- Wallace, David, 2012, The Emergent Multiverse: Quantum Theory According to the Everett Interpretation , Oxford: Oxford University Press. doi:10.1093/acprof:oso/9780199546961.001.0001
- Wasserman, Ryan, 2018, Paradoxes of Time Travel , Oxford: Oxford University Press. doi:10.1093/oso/9780198793335.001.0001
- Weyl, Hermann, 1918/1920 [1922/1952], Raum, Zeit, Materie , Berlin: Springer; fourth edition 1920. Translated as Space—Time—Matter , Henry Leopold Brose (trans.), New York: Dutton, 1922. Reprinted 1952, New York: Dover Publications.
- Wheeler, John Archibald and Richard Phillips Feynman, 1949, “Classical Electrodynamics in Terms of Direct Interparticle Action”, Reviews of Modern Physics , 21(3): 425–433. doi:10.1103/RevModPhys.21.425
- Yurtsever, Ulvi, 1990, “Test Fields on Compact Space‐times”, Journal of Mathematical Physics , 31(12): 3064–3078. doi:10.1063/1.528960
How to cite this entry . Preview the PDF version of this entry at the Friends of the SEP Society . Look up topics and thinkers related to this entry at the Internet Philosophy Ontology Project (InPhO). Enhanced bibliography for this entry at PhilPapers , with links to its database.
- Adlam, Emily, unpublished, “ Is There Causation in Fundamental Physics? New Insights from Process Matrices and Quantum Causal Modelling ”, 2022, arXiv: 2208.02721. doi:10.48550/ARXIV.2208.02721
- Rovelli, Carlo, unpublished, “ Can We Travel to the Past? Irreversible Physics along Closed Timelike Curves ”, arXiv: 1912.04702. doi:10.48550/ARXIV.1912.04702
causation: backward | determinism: causal | quantum mechanics | quantum mechanics: retrocausality | space and time: being and becoming in modern physics | time machines | time travel
Copyright © 2023 by Christopher Smeenk < csmeenk2 @ uwo . ca > Frank Arntzenius Tim Maudlin
- Accessibility
Support SEP
Mirror sites.
View this site from another server:
- Info about mirror sites
The Stanford Encyclopedia of Philosophy is copyright © 2023 by The Metaphysics Research Lab , Department of Philosophy, Stanford University
Library of Congress Catalog Data: ISSN 1095-5054
- Skip to main content
- Keyboard shortcuts for audio player

- LISTEN & FOLLOW
- Apple Podcasts
- Google Podcasts
- Amazon Music
- Amazon Alexa
Your support helps make our show possible and unlocks access to our sponsor-free feed.
Paradox-Free Time Travel Is Theoretically Possible, Researchers Say

Matthew S. Schwartz

A dog dressed as Marty McFly from Back to the Future attends the Tompkins Square Halloween Dog Parade in 2015. New research says time travel might be possible without the problems McFly encountered. Timothy A. Clary/AFP via Getty Images hide caption
A dog dressed as Marty McFly from Back to the Future attends the Tompkins Square Halloween Dog Parade in 2015. New research says time travel might be possible without the problems McFly encountered.
"The past is obdurate," Stephen King wrote in his book about a man who goes back in time to prevent the Kennedy assassination. "It doesn't want to be changed."
Turns out, King might have been on to something.
Countless science fiction tales have explored the paradox of what would happen if you went back in time and did something in the past that endangered the future. Perhaps one of the most famous pop culture examples is in Back to the Future , when Marty McFly goes back in time and accidentally stops his parents from meeting, putting his own existence in jeopardy.
But maybe McFly wasn't in much danger after all. According a new paper from researchers at the University of Queensland, even if time travel were possible, the paradox couldn't actually exist.
Researchers ran the numbers and determined that even if you made a change in the past, the timeline would essentially self-correct, ensuring that whatever happened to send you back in time would still happen.
"Say you traveled in time in an attempt to stop COVID-19's patient zero from being exposed to the virus," University of Queensland scientist Fabio Costa told the university's news service .
"However, if you stopped that individual from becoming infected, that would eliminate the motivation for you to go back and stop the pandemic in the first place," said Costa, who co-authored the paper with honors undergraduate student Germain Tobar.
"This is a paradox — an inconsistency that often leads people to think that time travel cannot occur in our universe."
A variation is known as the "grandfather paradox" — in which a time traveler kills their own grandfather, in the process preventing the time traveler's birth.
The logical paradox has given researchers a headache, in part because according to Einstein's theory of general relativity, "closed timelike curves" are possible, theoretically allowing an observer to travel back in time and interact with their past self — potentially endangering their own existence.
But these researchers say that such a paradox wouldn't necessarily exist, because events would adjust themselves.
Take the coronavirus patient zero example. "You might try and stop patient zero from becoming infected, but in doing so, you would catch the virus and become patient zero, or someone else would," Tobar told the university's news service.
In other words, a time traveler could make changes, but the original outcome would still find a way to happen — maybe not the same way it happened in the first timeline but close enough so that the time traveler would still exist and would still be motivated to go back in time.
"No matter what you did, the salient events would just recalibrate around you," Tobar said.
The paper, "Reversible dynamics with closed time-like curves and freedom of choice," was published last week in the peer-reviewed journal Classical and Quantum Gravity . The findings seem consistent with another time travel study published this summer in the peer-reviewed journal Physical Review Letters. That study found that changes made in the past won't drastically alter the future.
Bestselling science fiction author Blake Crouch, who has written extensively about time travel, said the new study seems to support what certain time travel tropes have posited all along.
"The universe is deterministic and attempts to alter Past Event X are destined to be the forces which bring Past Event X into being," Crouch told NPR via email. "So the future can affect the past. Or maybe time is just an illusion. But I guess it's cool that the math checks out."
- time travel
- grandfather paradox
Time Travel Possible? What Scientists Found Out With Latest Experiment
The study was a gedankenexperiment, a term used by albert einstein to refer to theoretical investigations carried out in place of actual experiments which are helpful for probing the boundaries of physics..
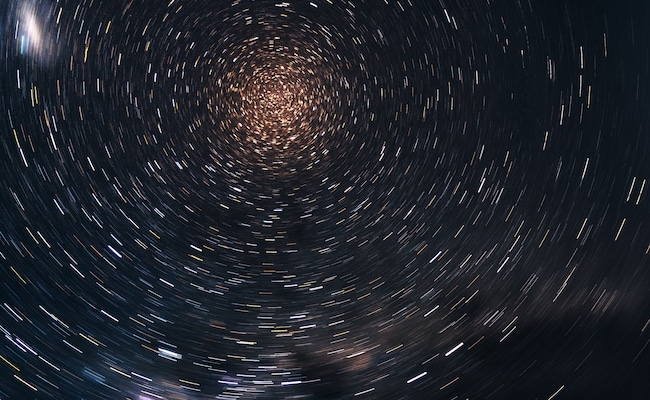
We all have a fascination with the idea of time travel, much like what we see in popular fiction. In films, we have mostly witnessed people sitting in a large, heavy machine and travelling hundreds of years into the past or future. However, this is not the case. Now scientists have come up with another experiment using quantum entanglement to simulate the activity- the strange way that quantum particles can interact, as per Gizmodo Newsletter . The concept found its way in the 2018 movie 'Ant-Man And The Wasp'.
The latest study was a Gedankenexperiment, a term used by Albert Einstein to refer to theoretical investigations carried out in place of actual experiments which are helpful for probing the boundaries of physics, such as particle motion at the speed of light. However, the team's latest work in Physical Review Letters describes a simulation that contains "effective time travel." The phenomenon wherein the properties of two or more quantum particles are determined by one another is known as quantum entanglement. Since the entanglement of two particles occurs on a quantum level, factors like their physical distance have no effect on the interaction. Hence, understanding the characteristics of one entangled particle provides knowledge about the other.
A possible time-travelling method known as closed-timelike curves was investigated in a recent study. The curve is the arc formed by a particle throughout its lifetime in spacetime that runs backwards. In the experiment, scientists subject photonic probes to a quantum interaction, with the aim of producing a quantifiable outcome.
They may ascertain what input would have produced the best outcome based on that outcome. However, since the outcome was the consequence of a quantum operation, the researchers may adjust the quantum probe's values through entanglement to get a better result even if the operation has already taken place, as opposed to being stuck with a less-than-ideal outcome, as per the Gizmodo Newsletter.
Promoted Listen to the latest songs, only on JioSaavn.com
Through the study, scientists found that the time travel effect would happen once every four times, or at a failure rate of 75 per cent. The researchers proposed delivering a lot of entangled photons and using a filter to verify that the photons with the revised information got through while sifting away the outdated particles in order to solve the high failure rate.
David Arvidsson-Shukur, a quantum physicist at the University of Cambridge and the study's author told the outlet, "The experiment that we describe seems impossible to solve with standard (not quantum) physics, which obeys the normal arrow of time. Thus, it appears as if quantum entanglement can generate instances which effectively look like time travel."
Track Budget 2023 and get Latest News Live on NDTV.com.
Track Latest News Live on NDTV.com and get news updates from India and around the world .
India Elections | Read Latest News on Lok Sabha Elections 2024 Live on NDTV.com . Get Election Schedule , information on candidates, in-depth ground reports and more - #ElectionsWithNDTV
Watch Live News:

Create a free profile to get unlimited access to exclusive videos, sweepstakes, and more!
How time travel gets weird: The science behind 'The Adam Project'
Time travel sounds stressful, honestly.
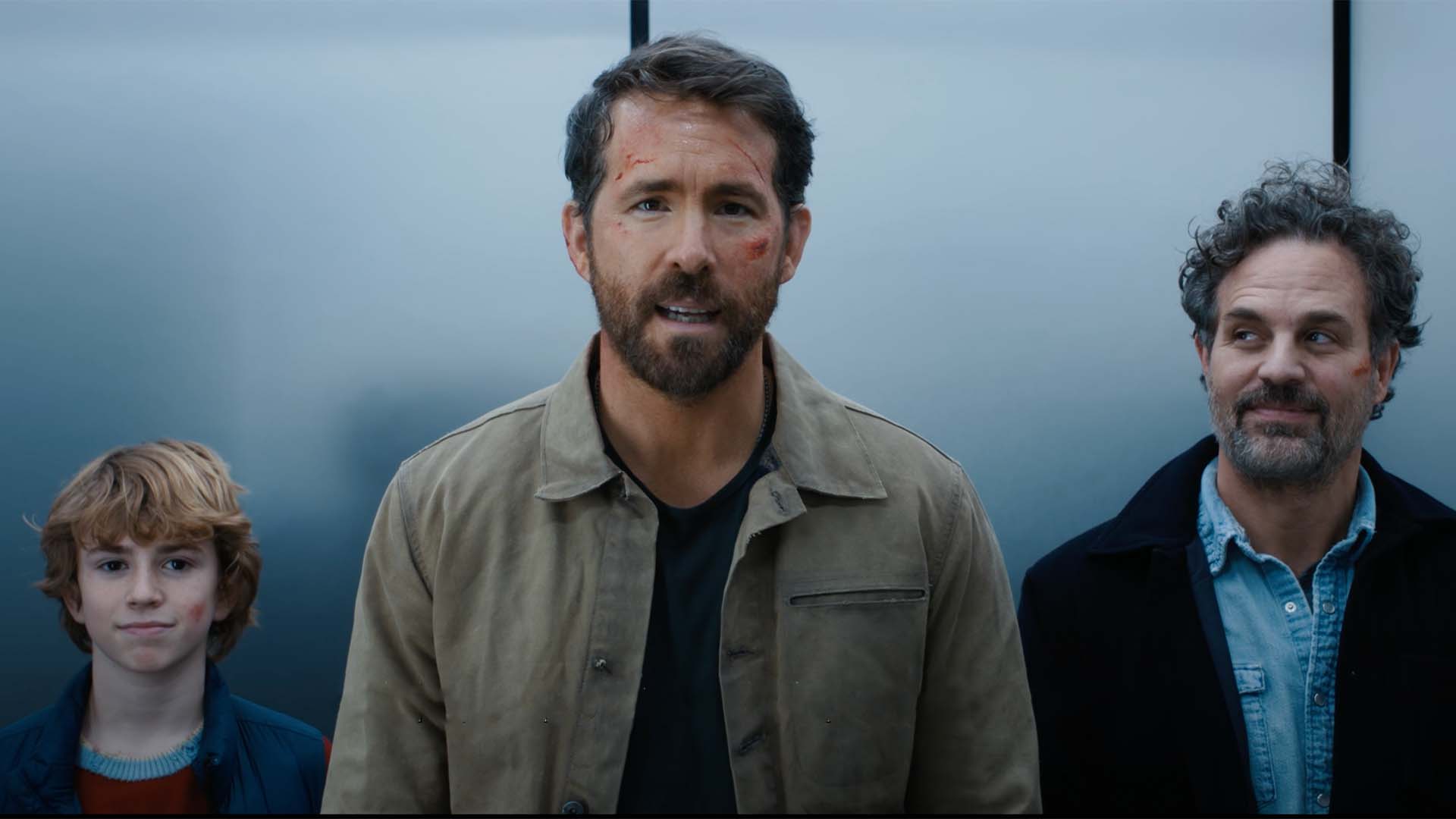
We love a good time travel story , we love a good family friendly flick without a trip to the theater, and we love Ryan Reynolds . Basically, The Adam Project hits all the marks we're looking for in these trying times. That said, it does play a little fast and loose with what we've come to understand about the rules of time travel. Fair warning, there are spoilers for the movie after this sentence.
Last chance to turn back before your own timeline is irrevocably changed.
Okay. Many time travel stories go to great lengths to avoid contaminating the timeline by interacting with your past self, but the plot of The Adam Project relies on those interactions. Adam, played by Ryan Reynolds, is a pilot from the year 2050 who comes back in time to the year 2022 where he teams up with his 12-year-old self to prevent the invention of time travel. He's basically doing a terminator but less violent and more attractive. Sorry, Arnold .
Meanwhile, Adam's boss has also been going back in time to give her younger self financial tips so she can amass a fortune and maintain control over the future. Because of some handwavey explanations — director Shawn Levy told SYFY WIRE that the time travel "rules" in The Adam Project are intentionally very simple — reality doesn't crumble into a pile of competing paradoxes, but it probably should.
THE GRANDFATHER PARADOX
Time more or less travels in a straight line. We could get into the wiggly bits to do with time dilation and the relative experience of time each of us has depending on our speed and point of view, but that's mostly irrelevant at the speeds we typically travel, so we can probably ignore it for now.
What is relevant is that effect follows after cause. The stream of events always flows in one way. Trinkets don't hit the fall and shatter until after they've fallen off the shelf. The arrow of time is linear. When we introduce an element of time travel things get more complicated.
Once a person goes back in time, the arrow of time is no longer linear, it's curved back on itself. Cause and effect falls apart, especially if the traveler comes into contact with their younger self or any of their direct ancestors. To that end, it's a decidedly bad idea to team up with your 12-year-old self but you might be able to get away with it as long as you don't do anything which would prevent you from going back in time in the first place.
That's where the Grandfather Paradox gets its name. It's a thought experiment in which a traveler goes back in time and, for whatever reason, decides to kill their own grandfather before their parent is born. You can come up with any number of similar scenarios but the crux of it relies on the traveler completing an action which prevents their ability to have been there at all. If you kill your grandfather then you'd never be born, then you couldn't go back in time, so you would be born, so you'd go back in time and kill your grandfather… you get the idea.
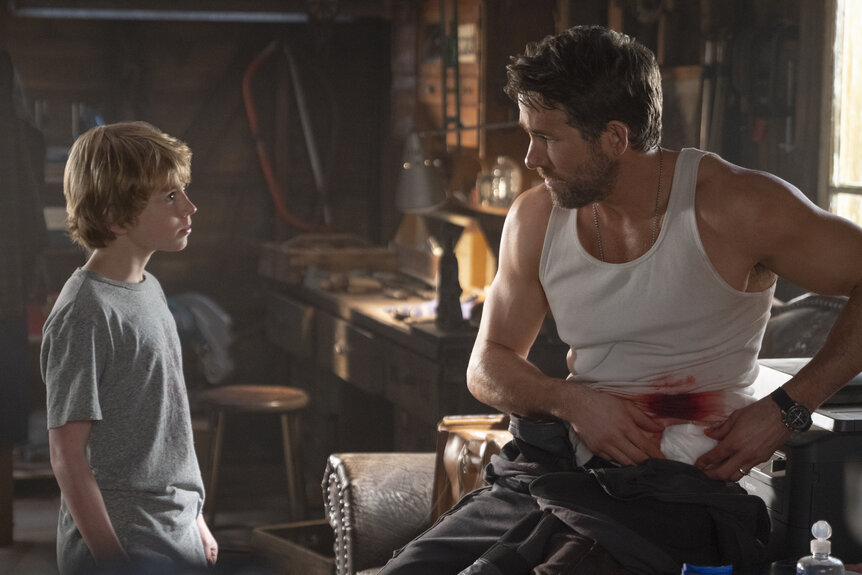
The Adams, young and old, travelling back to 2018 to stop their father from inventing time travel is the ultimate grandfather paradox. They're not killing anyone, but they're preventing not only themselves but anyone else, from being able to time travel. They shouldn't be able to pull it off because reality would get stuck in an endless buffering loop trying to figure out how to move forward with two competing futures. But that's not the only big paradox at play here.
THE BOOTSTRAP PARADOX
Adam isn't the only one going back in time to change things. His boss, Sorian, the villain of the flick, has also been meddling with time for her own benefit. In fact, they're only in charge of the dystopian future because they've been going back in time to give themselves information. This is almost a textbook example of the Bootstrap Paradox .
This paradox isn't as immediately obvious, but it runs into similar problems, defying a simple explanation. To get a clearer view of the weirdness, lets change the scenario.
Imagine you're at the library browsing the shelves when you come across a book with no author, no title, and no library label on the spine. Inside, you find the instructions for building a time machine. You take the book home and get to work but it isn't easy. It takes decades but eventually you figure out how to build a working machine. While you were working, you always wondered where the book came from, who put it there? So, you travel back to the year you found it. You arrive at the library hours before your past self found the book. When you go to the shelf, the book isn't there. So, you find a quiet spot and watch, waiting to see who delivers the book to its spot, but no one ever comes. Finally, you see your younger self enter the library and start browsing. They're almost to the spot and the book still isn't there. That's when it hits you.
You put the book there. You delivered the book to your past self. The question then becomes where the book came from. It seems to inherently exist, being constantly passed from one version of yourself to another.
That's basically what's going on with Sorian in the movie. Instead of a book about time travel, it's money and power which are being passed backward in an endless loop. Sorian doesn't become powerful if their future self doesn't give them inside knowledge. Their future self doesn't have inside knowledge unless they become powerful. How does the cycle begin?
It's paradoxes like these which make some scientists believe time travel to the past is impossible. Other scientists believe time travel is possible, but the paradoxes aren't. Maybe the universe prevents you from changing anything which would result in a paradox.
We may never know the truth. Or maybe we always have.
Watch SurrealEstate
- Science Behind The Fiction
- The Adam Project
- Time Travel
Related Stories
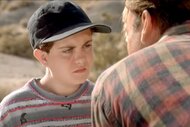
Jurassic Park's '6-Foot Turkey' Kid Looks Back on Iconic Role
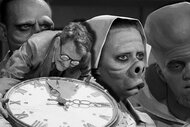
The 10 Best, Most Shocking, WTF Twilight Zone Twist Endings
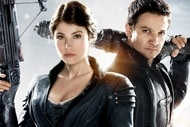
The Dark History Behind the Fairy Tale of Hansel and Gretel
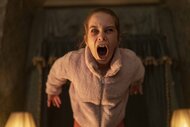
Abigail Sets Streaming Premiere: What to Know, How to Watch at Home
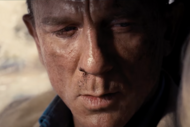
Are No Time to Die's Designer Diseases Possible in Real Life?
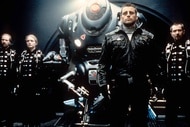
How 1990s Lost in Space Movie Was Meant to Launch a Franchise
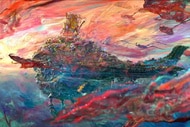
Deep Sea Animated Fantasy Film Streaming Now on Peacock
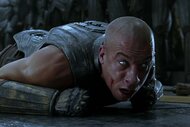
How The Chronicles of Riddick Turns a Simple Sci-Fi Story Into Dune
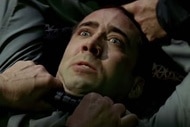
Face/Off Remains One of the Best & Wildest '90s Action Movies
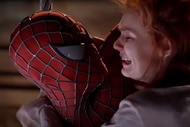
Kristen Dunst Reveals Dangerous Spider-Man Stunt She Refused
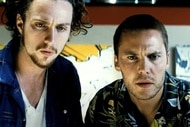
Savages: The Slept-on Oliver Stone Thriller with a Killer Cast
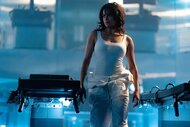
Michelle Rodriguez reflects on Fast Saga's hip-hop ethos
Recommended for you.
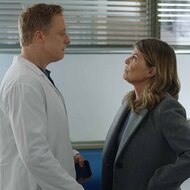
Linda Hamilton on Resident Alien Role: "I'm Not the Funny Girl, I'm the Straight Man"
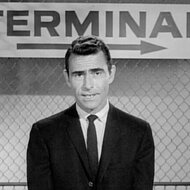
The Classic Twilight Zone Episode That Inspired Jordan Peele's Us
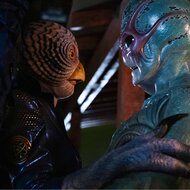
Resident Alien's Alan Tudyk on Harry's New Love Interest, Edi Patterson's Blue Avian
An astrophysicist claims he finally figured out time travel

If you buy through a BGR link, we may earn an affiliate commission, helping support our expert product labs.
Time travel has been one of the biggest tropes in science fiction for years. But what if you could actually go back in time and visit a loved one before their death? There’s, obviously, a lot we don’t know about what kind of consequences time travel might bring to the table , but that hasn’t stopped physicist Ron Mallet from a lifelong obsession with trying to figure out the time travel equation.
What’s even more impressive about this lifelong endeavor, though, is that Mallet now claims to have solved the equation and figured out how to build an actual time machine. Mallet’s inspiration and obsession with time travel originally began when he was much younger, Earth.com notes . Following the death of his beloved father, Mallet lost himself in novels, including The Time Machine by H.G. Wells.

It is certainly a respectable goal, especially for anyone who has lost someone they loved dearly. Mallet says that his idea of a time machine centers around an “intense and continuous rotating beam of light” that can manipulate gravity. A device built by him, following his equation, would use a ring of lasers to mimic the effects of a black hole, which appears to distort space and time around them.
Tech. Entertainment. Science. Your inbox.
Sign up for the most interesting tech & entertainment news out there.
By signing up, I agree to the Terms of Use and have reviewed the Privacy Notice.
Of course, learning the time travel equation and building a working time machine are two different things altogether. Sure, scientists have simulated black holes in a lab once or twice, but never anything with the kind of power or reality-effecting pull that Mallet seems to think would make time travel possible. That isn’t to say that he’s got things wrong, though.
His equation for time travel may be exactly what is needed to break through this lifelong obsession and actually travel back in time. But building something capable of testing it is going to be a whole separate endeavor in and of itself.
This article talks about:

Josh Hawkins has been writing for over a decade, covering science, gaming, and tech culture. He also is a top-rated product reviewer with experience in extensively researched product comparisons, headphones, and gaming devices.
Whenever he isn’t busy writing about tech or gadgets, he can usually be found enjoying a new world in a video game, or tinkering with something on his computer.
- Incredible medicine that regrows teeth is about to enter human trials
- New innovation may help EV batteries last significantly longer
- Northern Lights may be visible in these US states this weekend
More Science
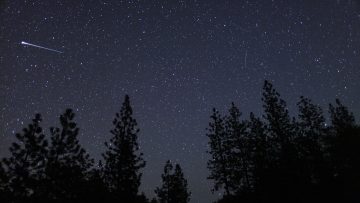
When to watch the Eta Aquariids meteor shower before it ends
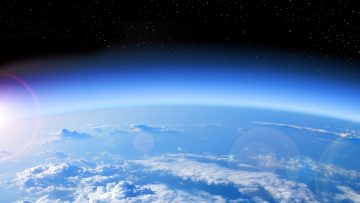
NOAA warns of possible severe geomagnetic storms this weekend
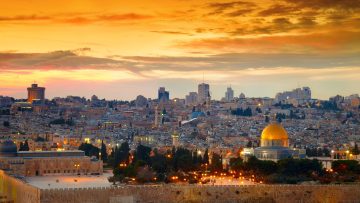
Some events in the Bible may have been confirmed by radiocarbon dating
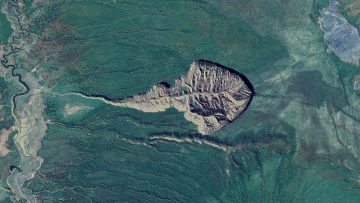
Siberia’s ‘gateway to the underworld’ won’t stop growing
Latest news.

Infinix Note 40 Pro+ review: Note quite there

I ran my first marathon wearing an Apple Watch SE and AirPods – here’s how it went

The biggest TV shows dominating Prime Video, Netflix, Hulu, Apple TV+, and Max right now

XGIMI HORIZON Ultra 4K projector review: The home-sized movie theater I always wanted
Sign up for the most interesting tech & entertainment news out there.
The Best, Most Realistic Movie About Time Travel Cost $7,000
"I will show you the most important thing that any living organism has ever witnessed."
The Big Picture
- Primer , created on a budget of $7,000, became a cult classic and won multiple awards.
- The film's complex plot, lack of exposition, and fast-paced editing can make it difficult to follow and understand.
- Primer 's low budget contributes to its realistic feel, making it relatable and believable for viewers.
If ever a film were brought into existence through the sheer force of one man's will, 2004's Primer is it. Over the course of three years, creator Shane Carruth (who was in his late 20s at the inception) wrote, directed, produced, edited, starred in, and wrote the score for the incredibly innovative, super-grounded sci-fi, all on a budget of $7,000. Primer would go on to win the Sundance Grand Jury Prize and Alfred P. Sloan Prize and become a beloved sci-fi cult classic.
Primer is the story of Aaron (Carruth) and Abe ( David Sullivan ), two engineers who accidentally stumble onto time travel while working on other projects in Aaron's garage. The time machine itself is a simple, cramped box made mostly of PVC — no shiny DeLorean , no dimension-hopping T.A.R.D.I.S. — but what makes this version of time travel unique is that in order to travel back in time, the traveler must spend an equivalent amount of time inside the box. In other words, to travel six hours into the past, you must spend six hours in the box.
Immediately after their discovery, Aaron and Abe are ultra careful not to influence the past during their brief trips through time, fearing consequences they can't even guess at. But eventually, the temptation to change history becomes too great, and Aaron in particular becomes obsessed with his ability to dictate the outcomes of events. This creates multiple complex, interwoven timelines and ultimately leads to a rift between the two characters.
Four friends/fledgling entrepreneurs, knowing that there's something bigger and more innovative than the different error-checking devices they've built, wrestle over their new invention.
'Primer' Can Be Tough To Follow
Primer is famously cerebral , refusing to hold the audience's hand at any point. The two leads speak in arcane physics jargon throughout because Carruth was adamant that the dialogue should sound authentic. The tight (and, at times, perhaps over-tuned) editing keeps Primer fast-paced; no one will catch every detail of the complicated plot the first time through . Primer is also very light on exposition — the characters often have long conversations about topics that the audience, at least at first, has no context for. Only in the last third of the movie does the voiceover dip in more heavily and start explaining things, though often cryptically.
Between the complexity of the story, the jargon, and the pacing, Primer can be a challenge to follow ; some critics have even called it "antagonistic" toward the audience. But that wasn't Carruth's goal. Instead, as Carruth told IndieWire , "the information is in there" to create a coherent story; it just might take multiple viewings for you to get it all. But unlike some modern filmmakers who don't trust their audience to make inferences and instead prefer to spell everything out, Carruth trusts not only his audience's intelligence, but their fortitude — perhaps a bit too much at times.
The 21st Century’s Best Time Travel Movie So Far Is a Low-Budget Rom-Com
If Primer was an unlikely hit, then its creator was an equally unlikely filmmaker. Carruth has a degree in math and started his career as an engineer before quitting to pursue writing. (This training would turn out to be useful in the course of writing the script, though Carruth had to learn physics jargon by reading graduate students' papers online .) With no formal background in filmmaking, he taught himself scriptwriting, cinematography, and storyboarding in the process of making Primer . Carruth said during his interview with IndieWire that he never planned on starring in the film; he cast himself as one of Primer 's leads only because he had difficulty finding an actor who played the part the way he wanted it , with subtlety rather than drama, and with his ultra-low budget , he worried that someone else might cut and run in the middle of shooting, leaving them stranded.
Perhaps surprisingly, the acting is one of Primer 's biggest strengths . Either Carruth or Sullivan (who's gone on to have a broad and successful TV career) is in every scene, so the film rests entirely on their shoulders. Both performances are subdued and understated, yet a great deal of subtext lies just beneath the surface.
How Was 'Primer' Made?
After months of rehearsals, shooting took place over five weeks around Dallas, where Carruth lived at the time. Primer was shot on super 16 film; Carruth said during his IndieWire interview that he had considered going digital, but in the early 2000s, the technology wasn't yet there to create the look he wanted. However, this meant there was no budget for multiple takes . Once shooting was complete, Carruth did the editing himself, again learning the process as he went along and often having to edit around lack of footage and continuity errors. He also composed the score, a task he said he'd enjoy handing off to someone else the next time around.
In some ways, the story of Primer mirrors the story of its creation. Before their break-through discovery, Aaron and Abe, like Shane Carruth, are cash-strapped; they even vandalize their own cars and refrigerators for parts in their quest to create something people will want to buy. Carruth, too, had to cut corners due to lack of funds , using friends' and family's houses and apartments for many of the locations, relying on ambient lighting and sunlight, filming only a single take for many scenes because he couldn't afford to waste film.
And like his character, Carruth claims to be a "control freak." Primer starts out following four engineers working on patents in Aaron's garage, but as soon as Aaron and Abe realize what they've stumbled onto, Aaron insists on cutting out their two unwitting colleagues rather than revealing the truth to them. He also doesn't let his wife in on the secret, to Abe's surprise. And Aaron is the first one tempted to use the time machine to change the past, leading to his falling out with Abe. Carruth performed every job in the course of making Primer partly because he couldn't afford to hire anyone else, but also because he couldn't stand to give up control , a quality that he doesn't apologize for, because, as he says during his IndieWire interview, "it’s important to feel strongly about the material you’re working on."
The Low Budget Gives 'Primer' a Documentary Feel
The result? Primer 's ultra-low budget contributes to its grounded realism — there are no Avengers: Endgame or even Back to the Future -style special effects, and the main characters never travel more than a few days into the past, so fancy set pieces are unnecessary. Instead, Primer feels incredibly real, even documentary-like (the fact that the actors clearly haven't had professional hairstyling or makeup and appear to be wearing their own clothes contributes to this). Watching Primer , you can actually believe that if two smart but ultimately ordinary guys happened upon time travel by accident, this is exactly how it would play out.
After Primer 's success at Sundance, it had a very limited theatrical release, playing in no more than 31 theaters at a time over the course of a few months yet still bringing in over $400,000 at the domestic box office . Primer 's DVD release the following year quickly vaulted it to cult classic status, particularly among science fiction aficionados. Its complexity contributes to its rewatchability : after your third, fourth, or tenth viewing, you'll still be catching details you haven't noticed before.
Primer isn't for the faint-of-heart film viewer; it asks more of its audience than most films, and gives less. But serious sci-fi lovers and fans of movies that make you think should consider it a must-watch — and don't feel bad if you have to Google "Primer ending explained" after the fact.
Primer is available to rent on Prime Video and Apple TV+ in the U.S.
Rent on Prime Video
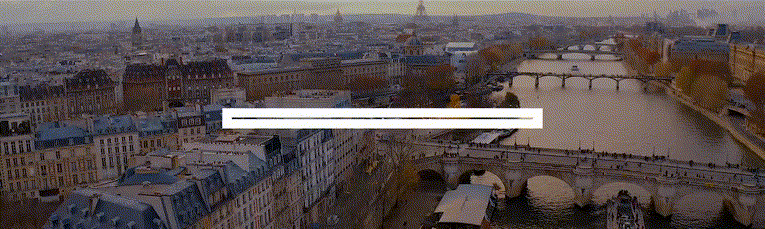
IMAGES
VIDEO
COMMENTS
Science says yes! This image from the Hubble Space Telescope shows galaxies that are very far away as they existed a very long time ago. ... the faster you travel, the slower you experience time. Scientists have done some experiments to show that this is true. For example, there was an experiment that used two clocks set to the exact same time ...
Science began to take time travel seriously in the 1980s. In 1990, for instance, Russian physicist Igor Novikov and American physicist Kip Thorne collaborated on a research paper about closed time ...
The Not-So-Distant Future of Human Time Travel. The Parker Solar Probe will reach speeds of 430,000 mph—fast but nowhere near the speed of light. Building a time-traveling spaceship may be the ...
The study cuts to the core of our understanding of the universe, and the resolution of the possibility of time travel, far from being a topic worthy only of science fiction, would have profound ...
Elementary School - Grades 4-6. P =Project E =Experiment. Build a water clock [ E] [ E] [ E] Black holes and time [ P] Does the temperature of water affect the time it takes for the water to freeze? [ E] Middle School - Grades 7-9. P =Project E =Experiment. Research the different methods of measuring time over history.
*Note: For this science project you will need to develop your own experimental procedure. Use the information in the summary tab as a starting place. ... A sidereal day is the time it takes for a particular star to travel around and reach same position in the sky. A sidereal day is slightly shorter than a mean day, lasting 23 hours, 56 minutes ...
Scientists are trying to figure out if time travel is even theoretically possible. If it is, it looks like it would take a whole lot more knowledge and resources than humans have now to do it.
According to NASA, time travel is possible, just not in the way you might expect. Albert Einstein's theory of relativity says time and motion are relative to each other, and nothing can go ...
A beginner's guide to time travel. Learn exactly how Einstein's theory of relativity works, and discover how there's nothing in science that says time travel is impossible. Everyone can travel in ...
Time travel, a longstanding fascination in science fiction, remains a complex and unresolved concept in science. The second law of thermodynamics suggests time can only move forward, while Einstein's theory of relativity shows time's relativity to speed. Theoretical ideas like wormholes offer potential methods, but practical challenges and ...
11 characters. Approximately ten minutes running time. Middle school students travel to a bleak future during the science fair. Fred and Dolly want to win a ribbon at the middle school science fair, so they built a time machine. When the students take a trip with their teacher into the future, they find humanity in danger and must now figure ...
Time travel and parallel timelines almost always go hand-in-hand in science fiction, but now we have proof that they must go hand-in-hand in real science as well. General relativity and quantum ...
Some physicists are convinced that time travel is possible. ... For as long as there has been science fiction, the concept of time travel has captured the imagination. ... "This is a project a ...
Physicists Just Figured Out How Wormholes Could Enable Time Travel. Physics 16 July 2023. By Mike McRae. (gremlin/Getty Images) Theoretical physicists have a lot in common with lawyers. Both spend a lot of time looking for loopholes and inconsistencies in the rules that might be exploited somehow. Valeri P. Frolov and Andrei Zelnikov from the ...
Time travel to the past is forbidden by some as-yet-undiscovered laws of physics. Physicists hope to discover a new theory that goes beyond general relativity and which explains why time loops are forbidden. We already have two possible candidates for such a theory, known as superstring theory and membrane theory.
Mallett was aged 10 when his father died suddenly, of a heart attack, an event that the scientist says changed the track of his life forever. "For me, the sun rose and set on him, he was just ...
The effect of time of day on the time it takes a hamster to negotiate a maze [ E] Change a mouse's internal clock. [ E] Which Saddle Pad Material Keeps the Horse at the Coolest Core Temperature After a Twenty Minute Workout [ E] Engineering. Build a water clock that tracks three hours of time. [ E]
Time Travel and Modern Physics. First published Thu Feb 17, 2000; substantive revision Mon Mar 6, 2023. Time travel has been a staple of science fiction. With the advent of general relativity it has been entertained by serious physicists. But, especially in the philosophy literature, there have been arguments that time travel is inherently ...
Bestselling science fiction author Blake Crouch, who has written extensively about time travel, said the new study seems to support what certain time travel tropes have posited all along. "The ...
Through the study, scientists found that the time travel effect would happen once every four times, or at a failure rate of 75 per cent. The researchers proposed delivering a lot of entangled ...
Many time travel stories go to great lengths to avoid contaminating the timeline by interacting with your past self, but the plot of The Adam Project relies on those interactions. Adam, played by Ryan Reynolds, is a pilot from the year 2050 who comes back in time to the year 2022 where he teams up with his 12-year-old self to prevent the ...
Of course, learning the time travel equation and building a working time machine are two different things altogether. Sure, scientists have simulated black holes in a lab once or twice, but never ...
Image by Annamaria Ward. The Big Picture. Primer, created on a budget of $7,000, became a cult classic and won multiple awards. The film's complex plot, lack of exposition, and fast-paced editing ...