Axons: the cable transmission of neurons
- Brain anatomy
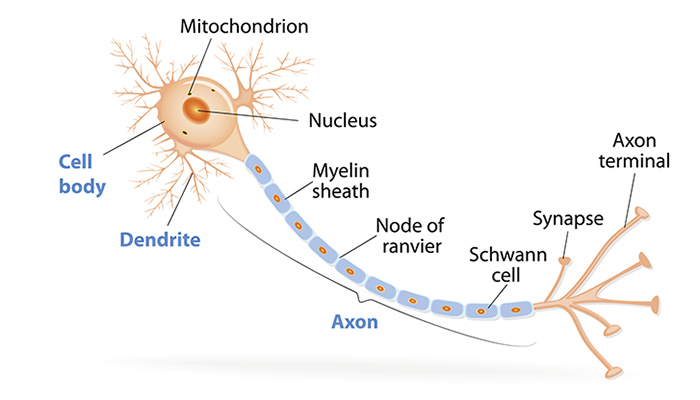
Each neuron in your brain has one long cable that snakes away from the main part of the cell. This cable, several times thinner than a human hair, is called an axon, and it is where electrical impulses from the neuron travel away to be received by other neurons.
Depending on the type of neuron, axons greatly vary in length - many are just a millimetre or so, but the longest ones, such as those that go from the brain down the spinal cord, can extend for more than a metre.
An axon typically develops side branches called axon collaterals, so that one neuron can send information to several others. These collaterals, just like the roots of a tree, split into smaller extensions called terminal branches. Each of these has a synaptic terminal on the tip.
Neurons communicate through synapses - contact points between the axon terminals on one side and dendrites or cell bodies on the other. Here, in a 20-40 nanometre-wide gap, electrical signals coming via the axon are converted into chemical signals through the release of neurotransmitters, and then promptly converted back into electricity as information moves from neuron to neuron.
Some axons are encased in a fatty substance called myelin, which is what makes your brain’s white matter white. Myelin acts as a form of insulation for axons, helping to send their signals over long distances. For this reason, myelin is mostly found in neurons that connect different brain regions, rather than in the neurons whose axons remain in the local region.
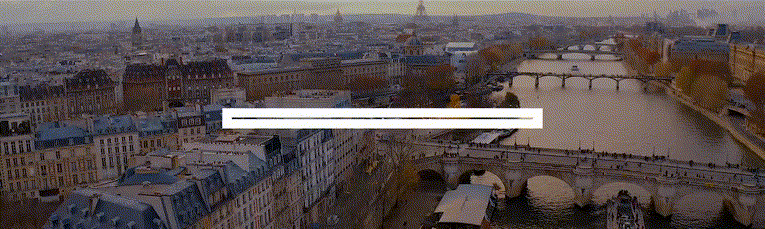
Axons and nerve degeneration
Neurons cannot properly communicate if axons are damaged or broken. This can happen both with nerve injury, and also in the earliest stages of neurodegenerative diseases such as motor neurone disease (MND), Alzheimer’s Disease and Parkinson’s Disease. Scientists at QBI are working to better understand the underlying processes and genetics involved.
Since axons are much longer than the rest of the cell, they need to be maintained by transporting essential molecules and organelles through them. QBI scientists have discovered that the gene mec-17 is involved in stabilising the internal neuronal structure to support proper transport within the axon and its maintenance. A mutation of this gene, and others with similar functions, can disrupt this process, leading to damaged axons and eventual disease.
QBI researchers have also discovered two proteins involved in axon degeneration in the roundworm C. elegans , a small organism which is an excellent research model for studying individual neurons and observing what happens at a molecular level. When an axon is damaged with a laser, it sends out signals to the surrounding tissue to be 'cleaned up', triggering the release of proteins that hastens degeneration of the axon. If such molecules are prevented from showing up, it could slow down the progress and extent of nerve damage.
Research that involves QBI scientists has also showed that severed neurons in roundworms ( C. elegans ) send out a 'save-me' signal to initiate nerve repair - to build a bridge to fuse the axon back together. This process was able to be modified by the researchers, giving hope for treating nerve injuries in humans in the future.
Image: istock
- Central Nervous System
- Lobes of the brain
- The spinal cord
- What is a neuron?
- Types of neurons
- Mitochondria
- Types of glia
- What are glia?
- Blood-brain barrier
- Corpus callosum
- The forebrain
- The hindbrain
- The midbrain
- The limbic system
- Peripheral Nervous System
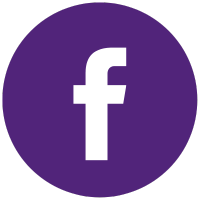
Help QBI research
Qbi newsletters.
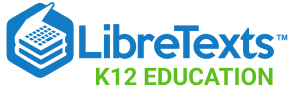
- school Campus Bookshelves
- menu_book Bookshelves
- perm_media Learning Objects
- login Login
- how_to_reg Request Instructor Account
- hub Instructor Commons
- Download Page (PDF)
- Download Full Book (PDF)
- Periodic Table
- Physics Constants
- Scientific Calculator
- Reference & Cite
- Tools expand_more
- Readability
selected template will load here
This action is not available.
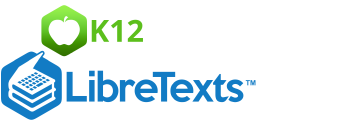
13.5: Nerve Impulse
- Last updated
- Save as PDF
- Page ID 13276
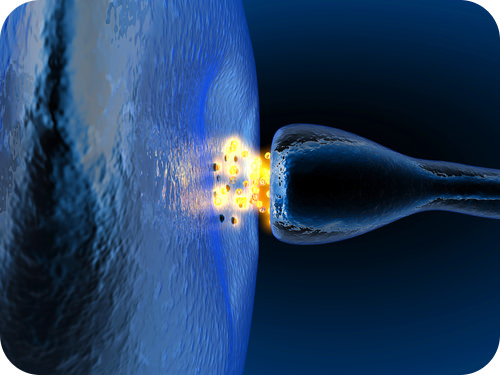
How does a nervous system signal move from one cell to the next?
It literally jumps by way of a chemical transmitter. Notice the two cells are not connected, but separated by a small gap. The synapse. The space between a neuron and the next cell.
Nerve Impulses
Nerve impulses are electrical in nature. They result from a difference in electrical charge across the plasma membrane of a neuron. How does this difference in electrical charge come about? The answer involves ions , which are electrically charged atoms or molecules.
Resting Potential
When a neuron is not actively transmitting a nerve impulse, it is in a resting state, ready to transmit a nerve impulse. During the resting state, the sodium-potassium pump maintains a difference in charge across the cell membrane (see Figure below). It uses energy in ATP to pump positive sodium ions (Na + ) out of the cell and potassium ions (K + ) into the cell. As a result, the inside of the neuron is negatively charged compared to the extracellular fluid surrounding the neuron. This is due to many more positively charged ions outside the cell compared to inside the cell. This difference in electrical charge is called the resting potential.
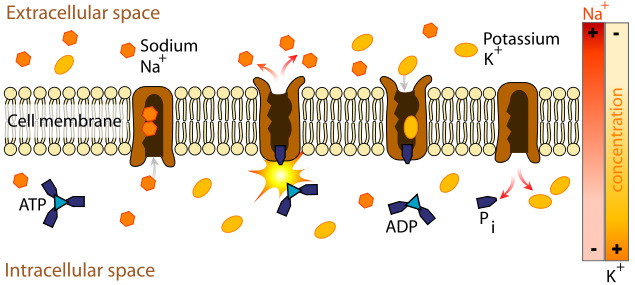
Action Potential
A nerve impulse is a sudden reversal of the electrical charge across the membrane of a resting neuron. The reversal of charge is called an action potential. It begins when the neuron receives a chemical signal from another cell. The signal causes gates in sodium ion channels to open, allowing positive sodium ions to flow back into the cell. As a result, the inside of the cell becomes positively charged compared to the outside of the cell. This reversal of charge ripples down the axon very rapidly as an electric current (see Figure below).
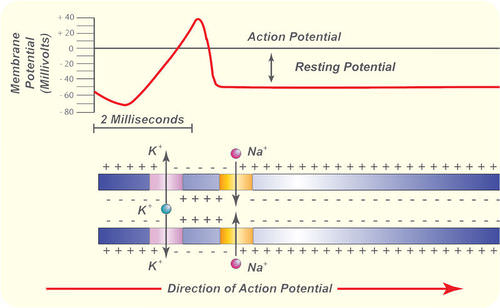
In neurons with myelin sheaths, ions flow across the membrane only at the nodes between sections of myelin. As a result, the action potential jumps along the axon membrane from node to node, rather than spreading smoothly along the entire membrane. This increases the speed at which it travels.
The place where an axon terminal meets another cell is called a synapse . The axon terminal and other cell are separated by a narrow space known as a synaptic cleft (see Figure below). When an action potential reaches the axon terminal, the axon terminal releases molecules of a chemical called a neurotransmitter . The neurotransmitter molecules travel across the synaptic cleft and bind to receptors on the membrane of the other cell. If the other cell is a neuron, this starts an action potential in the other cell.
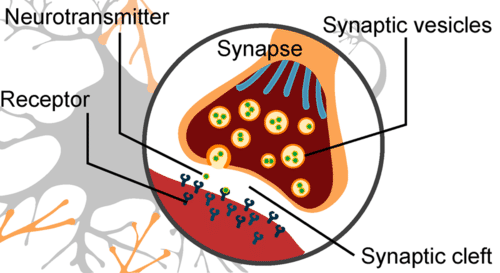
- A nerve impulse begins when a neuron receives a chemical stimulus.
- The nerve impulse travels down the axon membrane as an electrical action potential to the axon terminal.
- The axon terminal releases neurotransmitters that carry the nerve impulse to the next cell.
- Define resting potential and action potential.
- Explain how resting potential is maintained
- Describe how an action potential occurs.
- What is a synapse?

Want to create or adapt books like this? Learn more about how Pressbooks supports open publishing practices.
8.4 Nerve Impulses
Created by CK-12 Foundation/Adapted by Christine Miller
When Lightning Strikes
This amazing cloud-to-surface lightning occurred when a difference in electrical charge built up in a cloud relative to the ground. When the buildup of charge was great enough, a sudden discharge of electricity occurred. A nerve impulse is similar to a lightning strike. Both a nerve impulse and a lightning strike occur because of differences in electrical charge, and both result in an electric current.
Generating Nerve Impulses
A nerve impulse , like a lightning strike, is an electrical phenomenon. A nerve impulse occurs because of a difference in electrical charge across the plasma membrane of a neuron. How does this difference in electrical charge come about? The answer involves ions , which are electrically-charged atoms or molecules .
Resting Potential
When a neuron is not actively transmitting a nerve impulse, it is in a resting state, ready to transmit a nerve impulse. During the resting state, the sodium-potassium pump maintains a difference in charge across the cell membrane of the neuron. The sodium-potassium pump is a mechanism of active transport that moves sodium ions (Na+) out of cells and potassium ions (K+) into cells. The sodium-potassium pump moves both ions from areas of lower to higher concentration, using energy in ATP and carrier proteins in the cell membrane. The video below, “Sodium Potassium Pump” by Amoeba Sisters, describes in greater detail how the sodium-potassium pump works. Sodium is the principal ion in the fluid outside of cells, and potassium is the principal ion in the fluid inside of cells. These differences in concentration create an electrical gradient across the cell membrane, called resting potential . Tightly controlling membrane resting potential is critical for the transmission of nerve impulses.
Sodium Potassium Pump, Amoeba Sisters, 2020.
Action Potential
A nerve impulse is a sudden reversal of the electrical gradient across the plasma membrane of a resting neuron. The reversal of charge is called an action potential . It begins when the neuron receives a chemical signal from another cell or some other type of stimulus . If the stimulus is strong enough to reach threshold , an action potential will take place is a cascade along the axon.
This reversal of charges ripples down the axon of the neuron very rapidly as an electric current, which is illustrated in the diagram below (Figure 8.4.2). A nerve impulse is an all-or-nothing response depending on if the stimulus input was strong enough to reach threshold. If a neuron responds at all, it responds completely. A greater stimulation does not produce a stronger impulse.
In neurons with a myelin sheath on their axon, ions flow across the membrane only at the nodes between sections of myelin. As a result, the action potential appears to jump along the axon membrane from node to node, rather than spreading smoothly along the entire membrane. This increases the speed at which the action potential travels.
Transmitting Nerve Impulses
The place where an axon terminal meets another cell is called a synapse . This is where the transmission of a nerve impulse to another cell occurs. The cell that sends the nerve impulse is called the presynaptic cell , and the cell that receives the nerve impulse is called the postsynaptic cell .
Some synapses are purely electrical and make direct electrical connections between neurons. Most synapses, however, are chemical synapses. Transmission of nerve impulses across chemical synapses is more complex.
Chemical Synapses
At a chemical synapse, both the presynaptic and postsynaptic areas of the cells are full of molecular machinery that is involved in the transmission of nerve impulses. As shown in Figure 8.4.3, the presynaptic area contains many tiny spherical vessels called synaptic vesicles that are packed with chemicals called neurotransmitters . When an action potential reaches the axon terminal of the presynaptic cell, it opens channels that allow calcium to enter the terminal. Calcium causes synaptic vesicles to fuse with the membrane, releasing their contents into the narrow space between the presynaptic and postsynaptic membranes. This area is called the synaptic cleft . The neurotransmitter molecules travel across the synaptic cleft and bind to receptors , which are proteins embedded in the membrane of the postsynaptic cell.
Neurotransmitters and Receptors
There are more than a hundred known neurotransmitters, and more than one type of neurotransmitter may be released at a given synapse by a presynaptic cell. For example, it is common for a faster-acting neurotransmitter to be released, along with a slower-acting neurotransmitter. Many neurotransmitters also have multiple types of receptors to which they can bind. Receptors, in turn, can be divided into two general groups: chemically gated ion channels and second messenger systems.
- When a chemically gated ion channel is activated, it forms a passage that allows specific types of ions to flow across the cell membrane. Depending on the type of ion, the effect on the target cell may be excitatory or inhibitory .
- When a second messenger system is activated, it starts a cascade of molecular interactions inside the target cell. This may ultimately produce a wide variety of complex effects, such as increasing or decreasing the sensitivity of the cell to stimuli, or even altering gene transcription.
The effect of a neurotransmitter on a postsynaptic cell depends mainly on the type of receptors that it activates, making it possible for a particular neurotransmitter to have different effects on various target cells. A neurotransmitter might excite one set of target cells, inhibit others, and have complex modulatory effects on still others, depending on the type of receptors. However, some neurotransmitters have relatively consistent effects on other cells. Consider the two most widely used neurotransmitters, glutamate and GABA (gamma-aminobutyric acid). Glutamate receptors are either excitatory or modulatory in their effects, whereas GABA receptors are all inhibitory in their effects in adults.
Problems with neurotransmitters or their receptors can cause neurological disorders. The disease myasthenia gravis , for example, is caused by antibodies from the immune system blocking receptors for the neurotransmitter acetylcholine in postsynaptic muscle cells. This inhibits the effects of acetylcholine on muscle contractions, producing symptoms, such as muscle weakness and excessive fatigue during simple activities. Some mental illnesses (including depression ) are caused, at least in part, by imbalances of certain neurotransmitters in the brain. One of the neurotransmitters involved in depression is thought to be serotonin , which normally helps regulate mood, among many other functions. Some antidepressant drugs are thought to help alleviate depression in many patients by normalizing the activity of serotonin in the brain.
8.4 Summary
- A nerve impulse is an electrical phenomenon that occurs because of a difference in electrical charge across the plasma membrane of a neuron.
- The sodium-potassium pump maintains an electrical gradient across the plasma membrane of a neuron when it is not actively transmitting a nerve impulse. This gradient is called the resting potential of the neuron.
- An action potential is a sudden reversal of the electrical gradient across the plasma membrane of a resting neuron. It begins when the neuron receives a chemical signal from another cell or some other type of stimulus. The action potential travels rapidly down the neuron’s axon as an electric current and occurs in three stages: Depolarization, Repolarization and Recovery.
- A nerve impulse is transmitted to another cell at either an electrical or a chemical synapse . At a chemical synapse, neurotransmitter chemicals are released from the presynaptic cell into the synaptic cleft between cells. The chemicals travel across the cleft to the postsynaptic cell and bind to receptors embedded in its membrane.
- There are many different types of neurotransmitters. Their effects on the postsynaptic cell generally depend on the type of receptor they bind to. The effects may be excitatory, inhibitory, or modulatory in more complex ways. Both physical and mental disorders may occur if there are problems with neurotransmitters or their receptors.
8.4 Review Questions
- Define nerve impulse.
- What is the resting potential of a neuron, and how is it maintained?
- Explain how and why an action potential occurs.
- Outline how a signal is transmitted from a presynaptic cell to a postsynaptic cell at a chemical synapse.
- What generally determines the effects of a neurotransmitter on a postsynaptic cell?
- Identify three general types of effects that neurotransmitters may have on postsynaptic cells.
- Explain how an electrical signal in a presynaptic neuron causes the transmission of a chemical signal at the synapse.
- The flow of which type of ion into a neuron results in an action potential? How do these ions get into the cell? What does this flow of ions do to the relative charge inside the neuron compared to the outside?
- Name three neurotransmitters.
8.4 Explore More
Action Potentials, Teacher’s Pet, 2018.
TED Ed| What is depression? – Helen M. Farrell, Parta Learning, 2017.
5 Weird Involuntary Behaviors Explained!, It’s Okay To Be Smart, 2015.
Attributions
Figure 8.4.1
Lightening/ Purple Lightning, Dee Why by Jeremy Bishop on Unsplash is used under the Unsplash License (https://unsplash.com/license).
Figure 8.4.2
Action Potential by CNX OpenStax, Biology on Wikimedia Commons is used under a CC BY 4.0 (https://creativecommons.org/licenses/by/4.0/deed.en) license.
Figure 8.4.3
Chemical_synapse_schema_cropped by Looie496 created file (adapted from original from US National Institutes of Health, National Institute on Aging) is in the public domain (https://en.wikipedia.org/wiki/Public_domain).
Amoeba Sisters. (2020, January 29). Sodium potassium pump. YouTube. https://www.youtube.com/watch?v=7NY6XdPBhxo&feature=youtu.be
CNX OpenStax. (2016, May 27) Figure 4 The action potential is conducted down the axon as the axon membrane depolarizes, then repolarizes [digital image]. In Open Stax, Biology (Section 35.2). OpenStax CNX. https://cnx.org/contents/[email protected]:cs_Pb-GW@6/How-Neurons-Communicate
It’s Okay To Be Smart. (2015, January 26). 5 Weird involuntary behaviors explained! YouTube. https://www.youtube.com/watch?v=ZE8sRMZ5BCA&feature=youtu.be
Mayo Clinic Staff. (n.d.). Depression (major depressive disorder) [online article]. MayoClinic.org. https://www.mayoclinic.org/diseases-conditions/depression/symptoms-causes/syc-20356007
Mayo Clinic Staff. (n.d.). Myasthenia gravis [online article]. MayoClinic.org. https://www.mayoclinic.org/diseases-conditions/myasthenia-gravis/symptoms-causes/syc-20352036
National Institute on Aging. (2006, April 8). Alzheimers disease: Unraveling the mystery. National Institutes of Health. https://www.nia.nih.gov/ ( archived version )
Parta Learning. (2017, December 8). TED Ed| What is depression? – Helen M. Farrell. YouTube. https://www.youtube.com/watch?v=rBcU_apy0h8&t=291s
Teacher’s Pet. (2018, August 26). Action potentials. YouTube. https://www.youtube.com/watch?v=FEHNIELPb0s&feature=youtu.be
A signal transmitted along a nerve fiber.
An atom or molecule with a net electric charge due to the loss or gain of one or more electrons.
The smallest particle of an element that still has the properties of that element.
A molecule is an electrically neutral group of two or more atoms held together by chemical bonds.
A functional unit of the nervous system that transmits nerve impulses; also called a nerve cell.
A solute pump that pumps potassium into cells while pumping sodium out of cells, both against their concentration gradients. This pumping is active and occurs at the ratio of 2 potassium for every 3 calcium.
The semipermeable membrane surrounding the cytoplasm of a cell.
The movement of ions or molecules across a cell membrane into a region of higher concentration, assisted by enzymes and requiring energy.
A complex organic chemical that provides energy to drive many processes in living cells, e.g. muscle contraction, nerve impulse propagation, and chemical synthesis. Found in all forms of life, ATP is often referred to as the "molecular unit of currency" of intracellular energy transfer.
The difference in electrical charge across the plasma membrane of a neuron that is not actively transmitting a nerve impulse.
Reversal of electrical charge across the membrane of a resting neuron that travels down the axon of the neuron as a nerve impulse.
Something that triggers a behavior or other response.
The critical level to which a membrane potential must be depolarized to initiate an action potential.
The place where the axon terminal of a neuron transmits a chemical or electrical signal to another cell.
The cell that sends the nerve impulse.
The cell that receives the nerve impulse.
These membrane-bound organelles store various neurotransmitters that are released at the synapse. The release is regulated by a voltage-dependent calcium channel. Vesicles are essential for propagating nerve impulses between neurons and are constantly recreated by the cell.
A type of chemical that transmits signals from the axon of a neuron to another cell across a synapse.
A space that separates two neurons. It forms a junction between two or more neurons and helps nerve impulse pass from one neuron to the other.
A protein on a cell membrane or inside of a cell that binds with a hormone, neurotransmitter, or other chemical signal to produce a response.
A neurotransmitter that will have excitatory effects on the neuron, meaning it will increase the likelihood that a neuron will fire an action potential.
A neurotransmitter that decreases the likelihood that a neuron will fire an action potential.
A chemical that nerve cells use to send signals to other cells. It is by a wide margin the most abundant excitatory neurotransmitter in the vertebrate nervous system.
A naturally occurring amino acid that works as a neurotransmitter in your brain. Neurotransmitters function as chemical messengers. GABA is considered an inhibitory neurotransmitter because it blocks, or inhibits, certain brain signals and decreases activity in your nervous system.
An antibody, also known as an immunoglobulin, is a large, Y-shaped protein produced mainly by plasma cells that is used by the immune system to neutralize pathogens such as pathogenic bacteria and viruses.
An organic chemical that functions in the brain and body of many types of animals (and humans) as a neurotransmitter—a chemical message released by nerve cells to send signals to other cells, such as neurons, muscle cells and gland cells.
A neurotransmitter. It has a popular image as a contributor to feelings of well-being and happiness, though its actual biological function is complex and multifaceted, modulating cognition, reward, learning, memory, and numerous physiological processes such as vomiting and vasoconstriction.
Human Biology Copyright © 2020 by Christine Miller is licensed under a Creative Commons Attribution-NonCommercial 4.0 International License , except where otherwise noted.
Share This Book
- Type 2 Diabetes
- Heart Disease
- Digestive Health
- Multiple Sclerosis
- COVID-19 Vaccines
- Occupational Therapy
- Healthy Aging
- Health Insurance
- Public Health
- Patient Rights
- Caregivers & Loved Ones
- End of Life Concerns
- Health News
- Thyroid Test Analyzer
- Doctor Discussion Guides
- Hemoglobin A1c Test Analyzer
- Lipid Test Analyzer
- Complete Blood Count (CBC) Analyzer
- What to Buy
- Editorial Process
- Meet Our Medical Expert Board
Understanding the Structure and Function of an Axon
The Transmission Cable of Neurons
- Structure and Types
Function of Axons
Causes of axons injury.
Axons are thin fibers that enable communications between neurons (nerve cells). The function of axons is to transmit information in the form of electrical impulses between neurons.
From the broadest perspective, axons act like transmission cables. They enable the passage of electrical impulses within the brain and between the brain and the rest of the body.
The article looks at the structure, function, and types of axons in the body's nervous system . It also describes conditions that can damage axons and lead to nerve dysfunction.
koto_feja / Getty Images
Structure and Types of Axons
With a few exceptions, every neuron in the nervous system has only one axon. They come in different sizes and lengths.
Some axons are as long as one meter, while others are less than one millimeter. The longest are those of the sciatic nerve which run from the base of the spinal cord down each leg and end at your big toes.
As a general rule, the larger the diameter of the axon, the more quickly it can transmit (conduct) nerve impulses.
There are two types of axons found in the nervous system:
- Myelinated axons : These axons are covered with a fatty insulated coating called a myelin sheath . Myelinated axons connect neurons in the somatic nervous system which directs the voluntary movement of skeletal muscles in the body.
- Unmyelinated axons : These axons are not covered with a myelin sheath. Unmyelinated axons connect neurons in the autonomic nervous system which direct the involuntary movement of smooth muscles , like those of the heart, blood vessels, and intestines.
Myelinated and unmyelinated axons differ in how they work (and why).
Myelinated axons service the peripheral nervous system . This is part of the nervous system that carries nerve signals from your brain to skeletal (voluntary) muscles so that you can move. Nerve impulses move faster with myelinated axons, enabling rapid and intricate variations in movement.
Unmyelinated axons service the autonomic nervous system . This is part of the nervous system that regulates smooth (involuntary) muscles involved with things like heart rate, blood pressure, respiration, and digestion. Nerve impulses move slower with unmyelinated axons, enabling steady, consistent movement with less variation.
The function of an axon is to transmit information between neurons and away from muscles and glands.
Each neuron has an axon that directly connects it with another neuron. There are three types of neurons it can connect to:
- Sensory neurons : These are nerve cells that relay information that allow us to hear, touch, smell, see, or feel things like temperature or pain.
- Motor neurons : These are nerve cells that direct muscle contractions and gland functions.
- Interneurons : These connect nerve cells to nerve cells within the same region of the brain or spinal cord. Neurons connected in this way create a network known as a neural circuit.
How Electrical Impulses Become Communication
The translation of electrical impulses into actual communication involves chemical messengers known as neurotransmitters . These are what neurons use to communicate with each other. It also involves the movement of electrically charged particles called ions across the neuron's membrane.
The process starts when a signal, also called an action potential, is sent from the neuron's cell body towards the axon. Along the surface of the axon membrane are channels that allow ions like sodium and potassium to enter or exit the neuron. When the signal from the cell body reaches the nearest sodium channels, these channels open and sodium ions flood the inside of that segment of the axon.
This change in concentration of ions also changes the membrane voltage, which leads to ion channels in the next segment of the axon opening. At the same time, the previous segment returns to its resting potential. These steps repeat along the length of the axon until the signal reaches the end of axon, called the axon terminal.
At the axon terminal, neurotransmitters stored within the axon terminal are released. The neurotransmitters then cross a small gap, called a synapse , and are received by the dendrites of the adjacent neuron.
Different neurotransmitters deliver different chemical messages.
A single axon can have many different branches, each with different neurotransmitters. Depending on which branches are fired, multiple messages can be delivered simultaneously and rapidly between neurons.
Axons vs. Dendrites
Dendrites are small branched extensions from a neuron that are similar to axons. Dendrites differ in they receive nerve impulses from other neurons and deliver the signals to the cell body. Axons carry nerve impulses from the cell body to other neurons.
Dendrites also differ in that one neuron can many have many dendrites. In most cases, there is only one axon per neuron.
Axons can be damaged directly and indirectly. When this happens, certain physiological or neurological functions can be impaired, either temporarily or permanently. The impairment may be localized to one part of the body or involve the body as a whole.
The causes of axonal injury can be broadly categorized as follows:
Brain and Spinal Cord Trauma
Head and spinal cord trauma can cause the compression or severing of axonal connections, referred to as traumatic axonal injury (TAI) of the brain or spinal cord. When the brain is affected, a coma and other neurological problems can occur. When the spinal cord is affected, weakness, chronic pain, paralysis, or urinary or fecal incontinence can occur.
Axonal Degeneration
Age can cause axons to gradually deteriorate. The same can occur with neurodegenerative diseases like Alzheimer’s disease , Huntington’s disease , Parkinson’s disease , and amyotrophic lateral sclerosis (ALS) , which tend to be aging-related.
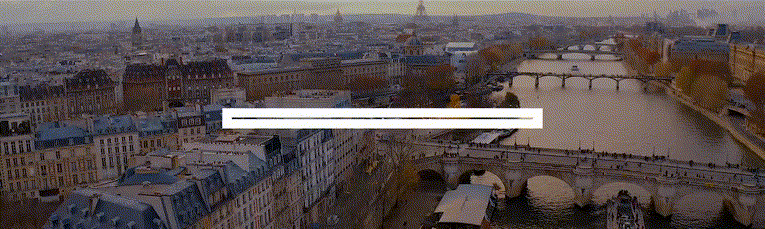
Axonal Demyelination
Diseases like multiple sclerosis (MS) cause the progressive destruction of the myelin sheath, resulting in the loss of motor function as axons "misfire." The loss of myelin is referred to as demyelination .
Diabetes and certain medications also cause demyelination, resulting in disruptive pain, burning, or tingling sensations known as neuropathy.
Metabolic Encephalopathy
Metabolic encephalopathies are conditions that cause chemical imbalances in the brain that lead to axonal inflammation and injury.
These include:
- Acute metabolic encephalopathy : Caused by a deficiency of vitamins, oxygen, or glucose
- Toxic metabolic encephalopathy : Caused by toxic substances or the accumulation of toxins from failing organs (such as kidney failure or liver failure)
Brain Infections
Certain brain infections can cause axonal damage, either acute (sudden and severe) or chronic (persistent or recurrent). These include conditions like cerebral malaria which can lead to seizures, coma, or death, and AIDS dementia complex, a complication of advanced untreated HIV infection.
Ischemic Injury
This occurs when decreased blood flow to the brain starves neurons and axons of the oxygen and nutrients they need to survive. Ischemic stroke is an example of this, often causing irreparable brain damage.
Subcortical ischemic vascular dementia (SIVD) is a form of dementia associated with atherosclerosis in which the narrowing of the arteries causes progressive damage to axons in the deeper issues of the brain (called the white matter).
An axon is a thin fiber that connects neurons (nerve cells) to that they can communicate. Neurons communicate via electrical impulses that trigger the release of "chemical messengers" called neurotransmitters. Axons also transmit electrical impulses from muscle and gland cells to the brain.
Axons can be damaged by trauma, inflammation, infection, or reduced blood flow to the brain. Damage can also be caused to the protective coating of axons, called the myelin sheath, due to older age, disease, or toxic substances.
Sasaki T. The axon as a unique computational unit in neurons . Neurosci Res 2013 Feb;75(2):83-8. doi:10.1016/j.neures.2012.12.004
Du F, Cooper AJ, Thida T, Shinn AK, Cohen BM, Ongür D. Myelin and axon abnormalities in schizophrenia measured with magnetic resonance imaging techniques . Biol Psychiatry . 2013;74(6):451-457. doi:10.1016/j.biopsych.2013.03.003
Society for Neuroscience. Sending the right signals .
By Michelle Pugle Pulge is a freelance health writer focused on mental health content. She is certified in mental health first aid.
Ohio State nav bar
The Ohio State University
- BuckeyeLink
- Find People
- Search Ohio State
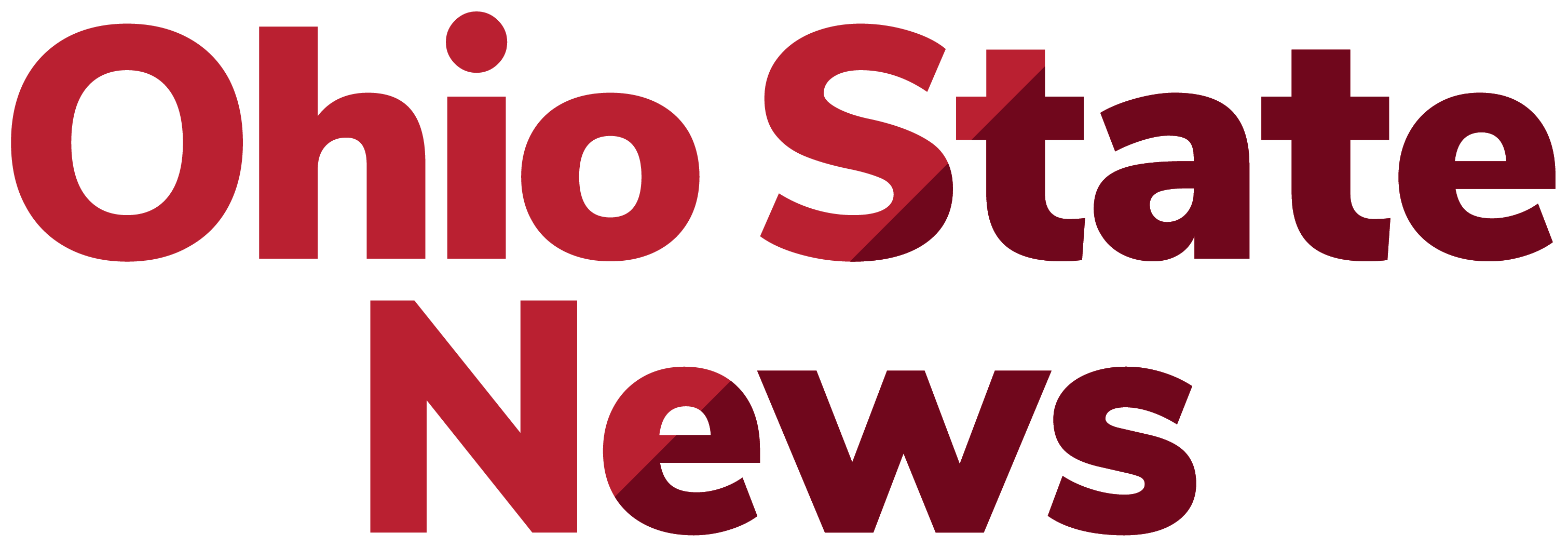
Mystery Solved: How Nerve Impulse Generators Get Where They Need to Go
This image of a neuron illustrates the movement of a nerve impulse along an axon – the long, slender extension of the nerve cell body. Ohio State University researchers have discovered how a key protein finds its way to the right spot on the axon to launch these vital electrical impulses, which enable communication of nerves’ sensory, movement and memory signals to and from the brain. Image courtesy of Chen Gu, Ohio State University
[Embargoed until 12 noon (ET) Thursday, Jan. 9, 2014]
Study identifies essential molecule for transport of protein from neuron cell body to axon
COLUMBUS, Ohio – Scientists have solved a longstanding mystery of the central nervous system, showing how a key protein gets to the right spot to launch electrical impulses that enable communication of nerve signals to and from the brain. Nerve impulses are critical because they are required for neurons to send information about senses, movement, thinking and feeling to other cell types in the neural circuitry. And an impulse is not fired up just once; it is initiated and then must be repeatedly transmitted along axons – long, slender extensions of nerve cell bodies – to keep the nervous system’s messages stable during their rapid travel.
For example, if your finger touches a hot stove, nerve impulses support quick communication between nerve cells in the hand and the brain so you avoid a serious burn.

This new research reveals a part of the process that was not understood before, about how a “molecular motor” helps move the impulse generator to its proper place on an axon to perform this vital job.
“This study is solving a very fundamental question,” said Chen Gu , assistant professor of neuroscience at The Ohio State University and lead author of the paper. “If these channel proteins don’t get into the nerves, nothing happens. They have to be correctly delivered and inserted into the axon to be functional.”
The research is published online in the journal Developmental Cell and will appear in the Jan. 27 print issue.
Like most proteins, the molecule that initiates nerve impulses is made in the cell body of a neuron, or nerve cell. But this protein, called a sodium ion channel , does its work on and between insulated segments of axons. Since nerve impulses were discovered in the 1950s, scientists have been unable to describe how the sodium channel gets to where it needs to be to initiate these electrical signals.
Previous research had shown that the sodium channel is anchored in axons through another protein. In this new study, Ohio State researchers identified a third molecule in the process: a motor protein that creates mechanical force to move the sodium channel and its partner protein from the cell body into the axon.
The research could help explain the deepest origins of many neurological disorders – ranging from multiple sclerosis and Parkinson’s disease to injuries of the spinal cord and brain – that are traced to malfunctioning or degenerated axons and the resulting improper electrical signaling.
“If the sodium channel can’t conduct the nerve impulse anymore, that gives rise to symptoms of neurological disorders.”
Gu is a longtime expert in research about the potassium ion channel , which is at the other end of a nerve impulse. This channel shuts off the signal, letting the axon rest and prepare for the next impulse to come along. His group has already gained deep understanding of how potassium channels get into the axon.
The research team accidentally found the binding between the anchor and motor proteins several years ago, which eventually led to the discovery of this transporting mechanism concerning the sodium channel. This channel is difficult to study because it is a large and complicated molecule relative to other players in the process.
The anchor protein, called ankyrin-G , is known to be tethered to sodium channel proteins once they reach axons. It is an adapter protein that helps other molecules connect, and is concentrated on axons close to the cell body as well as in regions called the nodes of Ranvier , which are gaps between axon segments (think of twisted casings between sausage links). These gaps are important to signal transmission because nerve impulses must jump across them to maintain the flow of communication.
The motor protein, called kinesin-1 , hooks up with ankyrin-G at the same time ankyrin-G is attached to the sodium channel. As a motor protein, kinesin-1 can produce mechanical force by making use of a specific energy source in cells. In an unusual scenario, both the sodium channel and kinesin-1 can connect with ankyrin-G at the same time using multiple binding sites.
“This allows ankyrin-G to bind to the sodium channel and the motor protein simultaneously so they can form a complex. Ankyrin-G plays its adapter role by loading the sodium channel onto the motor protein. Then the motor protein can act like a cargo carrier, transporting the sodium channel to the axon,” Gu said.
The researchers confirmed this process by observing the three proteins traveling together along an axon using live-cell imaging, as well as in studies using animals. Mice missing ankyrin-G in the cerebellum lacked the ability to move the sodium channels, and injecting a piece of the kinesin-1 molecule into the brains of normal mice interfered with the three-way protein interaction, also leaving the sodium channel proteins stuck in the cell body.
“We have identified protein-to-protein interactions between three very important and evolutionarily conserved molecules. We also found that if we damage the interaction using different strategies, the sodium channel won’t be transported,” Gu said.
“Mutations in the genes encoding the three proteins can lead to some neurological and mental disorders in humans. In many other diseases, the primary defect initiated by something else can alter the function of these three proteins – and particularly sodium channel transport and function – and ultimately disrupt the nerve impulse,” he said. “If the sodium channel can’t conduct the nerve impulse anymore, that gives rise to symptoms of neurological disorders.”
This work was mainly supported by grants from the National Institutes of Health , including the National Institute of Neurological Disorders and Stroke .
Gu is a faculty member in Ohio State’s Molecular, Cellular and Developmental Biology Graduate Program (MCDB) and Biomedical Sciences Graduate Program (BSGP). The authors of this study include Joshua Barry, an MCDB graduate student who is now in postdoc training at UCLA; and Yuanzheng Gu of the Department of Neuroscience; Peter Jukkola of the BSGP; Brian O’Neill, Howard Gu and Keerthi Thirtamara Rajamani of the Department of Pharmacology ; and Peter Mohler of the Davis Heart and Lung Research Institute and the departments of Internal Medicine and Physiology and Cell Biology , all at Ohio State.
Contact: Chen Gu, (614) 292-0349; [email protected]
Written by Emily Caldwell, (614) 292-8310; [email protected]
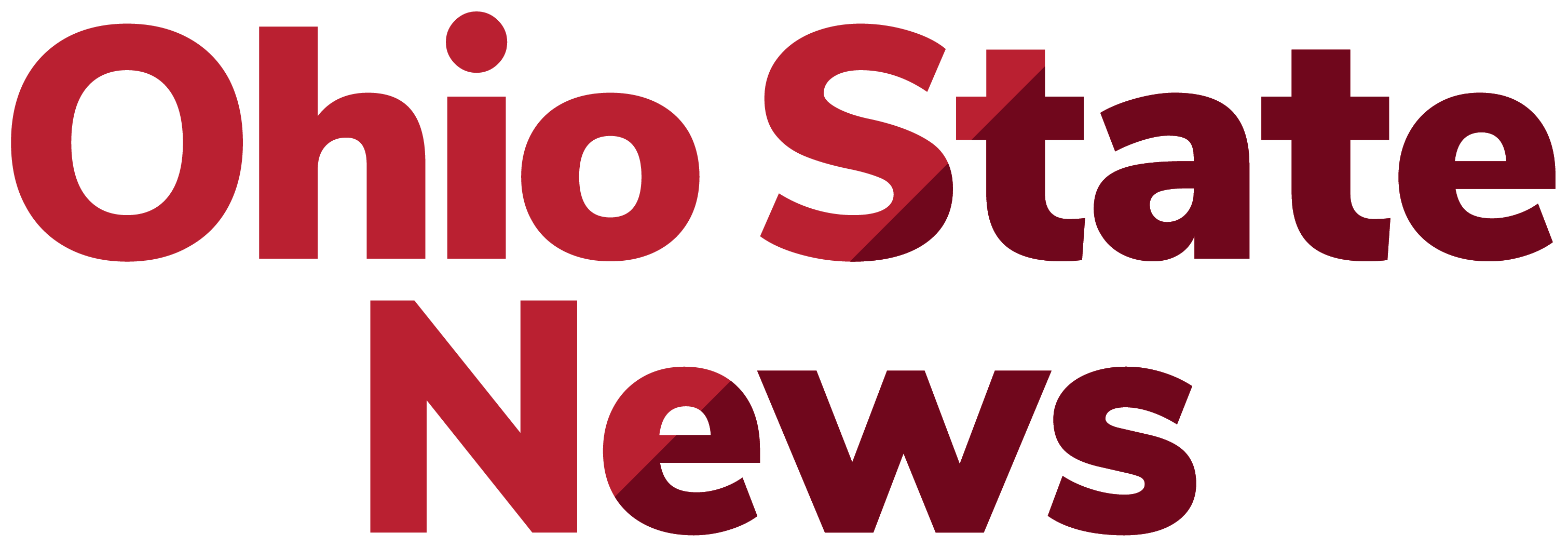
Contact: Admissions | Webmaster | Page maintained by University Communications
Request an alternate format of this page | Web Services Status | Nondiscrimination notice
A Level Biology
Instant Access to A Level Biology Revision
Sign up now to get access to the entire library of a level biology resources for all exam boards.
If you're ready to pass your A-Level Biology exams, become a member now to get complete access to our entire library of revision materials.
Join over 22,000 learners who have passed their exams thanks to us!
Sign up below to get instant access!
Or try a sample...
Not ready to purchase the revision kit yet? No problem. If you want to see what we offer before purchasing, we have a free membership with sample revision materials.
Signup as a free member below and you'll be brought back to this page to try the sample materials before you buy.
Nerve Impulse
Introduction, continuous conduction , saltatory conduction , resting membrane potential, action potential , polarization , depolarization, repolarization , refractory period , electrical synapses , chemical synapses , cns and nerve impulse, myelin sheath, axon diameter, temperature, what is a nerve impulse, how is a nerve impulse produced, what is the refractory period, what are saltatory impulses.
Nerve impulse was discovered by British Scientist Lord Adrian in the 1930s. Owning to the importance of this discovery, he was awarded Noble Prize in 1932. Nerve Impulse is a major mode of signal transmission for the Nervous system. Neurons sense the changes in the environment and as a result, generate nerve impulses to prepare the body against those changes.
A nerve Impulse is defined as a wave of electrical chemical changes across the neuron that helps in the generation of the action potential in response to the stimulus. This transmission of a nerve impulse across the neuron membrane as a result of a change in membrane potential is known as Nerve impulse conduction.
It is a change in the resting state of the neuron. Due to nerve impulses, the resting potential is changed to an action potential to conduct signals to the target in response to a stimulus. The stimulus can be a chemical, electrical, or mechanical signal.
The action potential is a result of the movement of ions in and out of the cell. Particularly the ions included in this process are sodium and potassium ions. These ions are propagated inside and outside the cell through specific sodium and potassium pumps present in the neuron membrane. The transmission of a nerve impulse from one neuron to another neuron is achieved by a synaptic connection (synapse) between them. It is thus a mode of communication between different cells.
The speed of nerve impulse propagation varies in different types of cells. The rate of transmission and generation of nerve impulses depends upon the type of cell. Besides, Myelin Sheath also helps in accelerating the rate of signal conduction (about 20 times). Generally, the speed of nerve impulses is 0.1-100 m/s.
Mechanism of Nerve Impulse Conduction
Nerve impulse conduction is a major process occurring in the body responsible for organized functions of the body. So, for the conduction of nerve impulses, there are two mechanisms:
- Continuous conduction
- Saltatory conduction
Continuous nerve impulse conduction occurs in non-myelinated axons. The action potential travels along the entire length of the axon. Hence, more time is taken in generating and then transmit nerve impulses during an action potential.
Continuous conduction requires more energy to transmit impulses and is a slower process (approximately 0.1 m/s). It delays the process of conducting signals because it uses a higher number of ion channels to alter the resting state of the neuron.
Saltatory is faster than continuous conduction and occurs in myelinated neurons. In myelinated neurons, myelinated sheaths are present. Between these myelinated sheaths, unmyelinated gaps are presently known as the nodes of Ranvier. Nerve impulse propagates by jumping from one node of Ranvier to the next. This makes the process of nerve impulse faster as the nerve impulse does not travel the entire length of the axon ( this happens in the case of continuous conduction). The nerve impulse travels at a speed of 100 m/s in saltatory conduction.
The number of channels utilized in saltatory conduction is less than in continuous conduction due to which delay of nerve impulse does not occur. This mode of nerve impulse transmission utilizes less energy as well.
If you consider the axon as an electrical wire or loop, nerve impulse that travels along the axon as current, and the charged particles ( sodium and potassium ions) as the electron particles then the process can be understood quite easily. As the flow of current in a wire occurs at a specific voltage only, similarly the conduction of nerve impulse occurs when a stimulus has a maximum threshold value of -55 millivolts. This is essential for altering the resting membrane state to action membrane potential.
When the voltage has the required number of electron particles it conducts current. Similarly, in the case of nerve impulse conduction, the neurons of the stimulus must have a threshold value for causing the movement of ions across the length of the axon (for conducting nerve impulse) by opening the voltage-gated ion channels.
Process of transmission of Nerve Impulse
For the transmission of a nerve impulse, the stages are below:
- Polarization
- Repolarization
- Refractory Period
Before going into the details of the process of nerve impulse transmission, let’s first discuss action and resting potential states.
The resting membrane potential refers to the non-excited state of the nerve cell at rest when no nerve impulse is being conducted. The resting membrane potential of the nerve cell is -70 mV. It is a static state and both the sodium and potassium channels are closed during this state maintaining a high concentration of sodium ions outside and high potassium ions concentration inside the cell.
An action potential occurs when the nerve cell is in an excited state while conducting nerve impulses. In this situation, sodium channels open and potassium channels are closed. This results in a huge influx of sodium ions inside the cells which trigger the nerve impulse conduction. The action potential is +40 mV.
Polarization is the situation in which the membrane is electrically charged but non-conductive. It means it doesn’t conduct nerve impulses in this state. During polarization, the membrane is in a resting potential state. The concentration of sodium ions is about 16 times more outside the axon than inside. In contrast, the concentration of potassium ions is 25 times more inside the axon than outside.
The polarization state is also known as the “Unstimulated or non-conductive state”. Due to the difference in the concentration of ions inside and outside the membrane, a potential gradient is established ranging between -20-200mV ( in the case of humans, the potential gradient in the polarized state is near -70mV). In the polarized state, the axon membrane is more permeable to potassium ions instead of sodium ions and as a result, it causes rapid diffusion of potassium ions.
In the resting state, the membrane potential becomes electro-negatively charged due to the movement of positively charged potassium ions outside the cell and the presence of electro-negative proteins in the intracellular space.
It refers to a graded potential state because a threshold stimulus of about -55mV causes a change in the membrane potential. The threshold stimulus must be strong enough to change the resting membrane potential into action membrane potential.
This results in the alternation in the electro-negativity of the membrane because the stimulus causes the influx of sodium ions (electropositive ions) by 10 times more than in the resting state. For this, sodium voltage-gated channels open. The action potential state is based on the “All or none” method and has two possibilities:
If the stimulus is not more than the threshold value, then there will be no action potential state across the length of the axon.
If the stimulus is more than the threshold value, then it will generate a nerve impulse that will travel across the entire length of the axon.
It is a condition during which the electrical balance is restored inside and outside the axon membrane. Due to the high concentration of sodium ions inside the axoplasm, the potassium channels will open. During the repolarization state, the efflux of potassium ions through the potassium channel occurs. As a result of the opening of potassium voltage-gated channels, sodium voltage-gated channels will be closed. Thus, no sodium ions will move inside the membrane. Therefore, repolarization helps in maintaining or restoring the original membrane potential state.
Until potassium channels close, the number of potassium ions that have moved across the membrane is enough to restore the initial polarized potential state. As a result of this, the membrane becomes hyperpolarized and has a potential difference of -90 mV.
The refractory phase is a brief period after the successful transmission of a nerve impulse. During this period, the membrane prepares itself for the conduction of the second stimulus after restoring the original resting state. It persists for only 2 milliseconds.
During this, the sodium ATPase pump allows the re-establishment of the original distribution of sodium and potassium ions. The sodium and potassium ATPase pump, driven by using ATP, helps to restore the resting membrane state for the conduction of a second nerve impulse in response to the other stimulus. It causes the movement of ions against the concentration gradient. For every two potassium ions that move inside the cell, three sodium ions are transported outside. This process requires ATP because the movement of ions is against the concentration gradient of both ions.
The process of transmission of a nerve impulse from one neuron to the other, after reaching the axon’s synaptic terminal, is known as synapse. This transmission of the nerve impulse by synapses involves the interaction between the axon ending of one neuron (Presynaptic neuron) to the dendrite of another neuron (Postsynaptic neuron). There is space between the pre-synaptic neuron and post-synaptic neuron which is known as synaptic cleft or synaptic gap.
After transmitting from one neuron to another, the nerve impulse generates a particular response after reaching the target site. If somehow the synaptic gap doesn’t allow the passage of nerve impulse, the transmission of nerve impulse will not occur and consequently required response too.
Read more about the Myelin Sheath
Types of synapses
There are two types of synapses:
- Electrical synapses
- Chemical synapses
In electrical synapses, two neurons are connected through channel proteins for transmitting a nerve impulse. The nerve impulse travels across the membrane of the axon in the form of an electrical signal. The signal is transmitted in the form of ions and therefore it is much faster than chemical synapses.
In electrical synapses, the synaptic gap is about 0.2nm which also favours faster nerve impulse conduction.
In chemical synapses, the conduction of nerve impulses occurs through chemical signals. These chemical signals are neurotransmitters. In this type of nerve impulse conduction, the synaptic gap is more than electrical synapses and is about 10-20 nm. Due to this, the transmission of nerve impulses is slower than electrical synapses.
Neurons help in transmitting signals in the form of nerve impulses from the Central nervous system to the peripheral body parts. Neurons are a complex network of fibres that transmit information from the axon ending of one neuron to the dendrite of another neuron. The signal finally reaches the target cell where it shows a response.
In conducting nerve impulses, the following play a major role:
- Axon- Helps in the propagation of nerve impulses to the target cell.
- Dendrites- Receive the signals from the axon ends.
- Axon Ending- Acts as a transmitter of signals.
Axon plays a major role in the process by transmitting signals in the form of nerve impulses via synapses to the target cells. The neuron is responsible for transferring signals to three target cells:
- Another neuron
And this results in the contraction of muscle, and secretion by glands and helps neurons to transmit action potential.
Factors Affecting the Speed of Nerve Impulse
The following are some major factors that affect the speed of nerve impulses:
Myelin sheath is present around the neuron and functions as an electrical insulator. Due to this sheath, an action potential is not formed on the surface of the neuron. This Myelin sheath has regular gaps, where it is not present, called nodes of Ranvier. An action potential can form at these gaps and impulse will jump from node to node by saltatory conduction. This can be a factor in increasing the speed of nerve impulses from about 30-1 m/ to 90-1 m/s.
As the axon diameter increase, the speed of nerve impulses increases as well. This is because a larger axon diminishes the ion leakage out of the axon. This helps in maintaining the membrane potential and thus favours faster nerve impulses.
Temperature cause changes in the rate of diffusion of ions across the neuron membrane. Temperature directly correlates with the transmission of nerve impulses. If the temperature is higher, the rate of diffusion of sodium and potassium ions will be high and the axon will become depolarized quickly which will cause a faster nerve impulse conduction.
A nerve impulse is thus an important signal transduction mode for triggering a response in major body parts due to a strong stimulus. Any distraction in this process can have drastic effects on the body.
Frequently Asked Questions
A nerve impulse is a wave of electrochemical changes that travel across the plasma membrane and helps in the generation of an action potential. Signals are propagated along the nerve fibres in the form of nerve impulses.
A nerve impulse is produced when a stimulus acts on the nerve fibre, resulting in electrochemical changes across the nerve membrane. These electrochemical changes cause depolarization of the membrane resulting in the generation of nerve impulses.
It is a short duration of time during which a new nerve impulse cannot be generated in a neuron, after initiation of a previous action potential. This period occurs at the end of action potential and limits the speed at which nerve impulses can be generated in a nerve fibre.
These are nerve impulses that jump from one node to another and are seen only in myelinated nerve fibres. Saltatory conduction increases the speed at which a nerve signal is conducted down the length of an axon.
- Lodish, H; Berk, A; Kaiser, C; Krieger, M; Bretscher, A; Ploegh, H; Amon, A (2000). Molecular Cell Biology (7th ed.). New York, NY: W. H. Freeman and Company. p. 695.
- Marieb, E. N., & Hoehn, K. (2014). Human anatomy & physiology. San Francisco, CA: Pearson Education Inc.
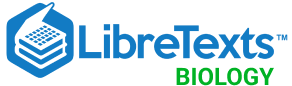
- school Campus Bookshelves
- menu_book Bookshelves
- perm_media Learning Objects
- login Login
- how_to_reg Request Instructor Account
- hub Instructor Commons
- Download Page (PDF)
- Download Full Book (PDF)
- Periodic Table
- Physics Constants
- Scientific Calculator
- Reference & Cite
- Tools expand_more
- Readability
selected template will load here
This action is not available.
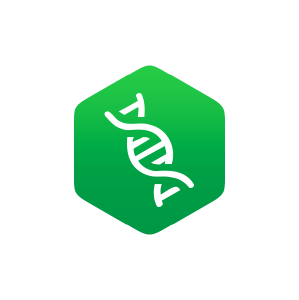
16.3: Neurons
- Last updated
- Save as PDF
- Page ID 92656
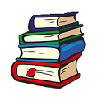
- Tara Jo Holmberg
- Northwestern Connecticut Community College
Life as Art
This colorful picture could be an abstract work of modern art. You might imagine it hanging in an art museum or art gallery. In fact, the picture illustrates real life rather than an artistic creation. It is a micrograph of human nervous tissue. The neon green structures in the picture are neurons. The neuron is one of two basic types of cells in the nervous system, the other type being the glial cell.
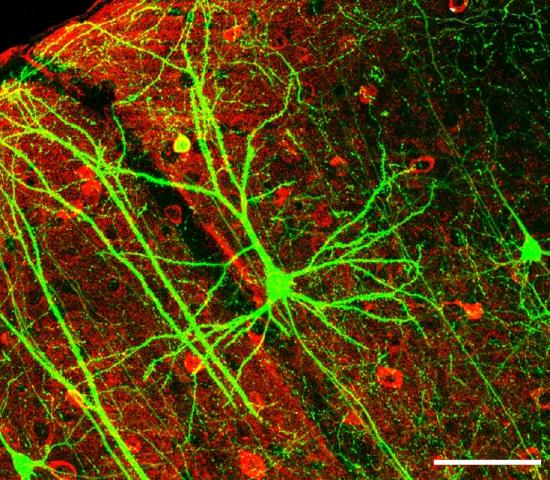
Neurons, also called nerve cells, are electrically excitable cells that are the main functional units of the nervous system. Their function is to transmit nerve impulses. They are the only type of human cells that can carry out this function.
Neuron Structure
Figure \(\PageIndex{2}\) shows the structure of a typical neuron. The main parts of a neuron are labeled in the figure and described below.
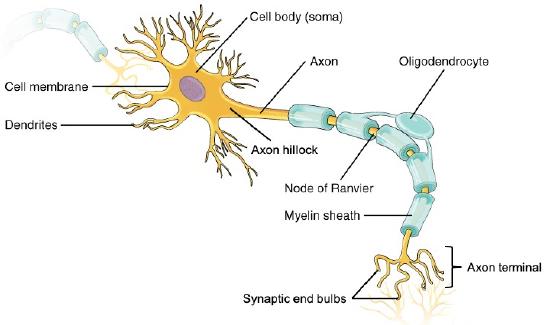
Figure \(\PageIndex{2}\): Somatic Motor Neuron with cell body, axon, axon, myelin sheath, nodes of Ranvier, axon terminal, dendrites, synaptic end of the bulbs, and other associated structures.
- The cell body is the part of a neuron that contains the cell nucleus and other cell organelles. It is usually quite compact, and may not be much wider than the nucleus.
- Dendrites are thin structures that are extensions of the cell body. Their function is to receive nerve impulses from other cells and carry them to the cell body. A neuron may have many dendrites, and each dendrite may branch repeatedly to form a dendrite “tree” with more than 1,000 “branches.” The end of each branch can receive nerve impulses from another cell, allowing a given neuron to communicate with tens of thousands of other cells.
- The axon is a long, thin extension of the cell body. It transmits nerve impulses away from the cell body and toward other cells. The axon branches at the end, forming multiple axon terminals. These are the points where nerve impulses are transmitted to other cells, often to the dendrites of other neurons. An area called a synapse occurs at each axon terminal. Synapses are complex membrane junctions that transmit signals to other cells. An axon may branch hundreds of times, but there is never more than one axon per neuron.
- Spread out along axons, especially the long axons of nerves, are many sections of the myelin sheath. These are lipid layers that cover sections of the axon. The myelin sheath is a very good electrical insulator, similar to the plastic or rubber that encases an electrical cord.
- Regularly spaced gaps between sections of myelin sheath occur along the axon. These gaps are called nodes of Ranvier , and they allow the transmission of nerve impulses along the axon. Nerve impulses skip from node to node, allowing nerve impulses to travel along the axon very rapidly.
- A Schwann cell (also on an axon) is a type of glial cell. Its function is to produce the myelin sheath that insulates axons in the peripheral nervous system. In the central nervous system, a different type of glial cell, called an oligodendrocyte, produces the myelin sheath.
Neurogenesis
Fully differentiated neurons, with all their special structures, cannot divide and form new daughter neurons. Until recently, scientists thought that new neurons could no longer be formed after the brain developed prenatally. In other words, they thought that people were born with all the brain neurons they would ever have, and as neurons died, they would not be replaced. However, new evidence shows that additional neurons can form in the brain, even in adults, from the division of undifferentiated neural stem cells that are found throughout the brain. The production of new neurons is called neurogenesis. The extent to which it can occur is not known, but it is not likely to be very great in humans.
Neurons in Nervous Tissues
The nervous tissue in the brain and spinal cord consists of gray matter and white matter. Gray matter contains mainly the cell bodies of neurons. It is gray only in cadavers; living gray matter is actually more pink than gray (see image below). White matter consists mainly of axons covered with myelin sheath, which gives them their white color. White matter also makes up nerves of the peripheral nervous system. Nerves consist of long bundles of myelinated axons that extend to muscles, organs, or glands throughout the body. The axons in each nerve are bundled together like wires in a cable. Axons in nerves may be more than a meter long in an adult. The longest nerve runs from the base of the spine to the toes.
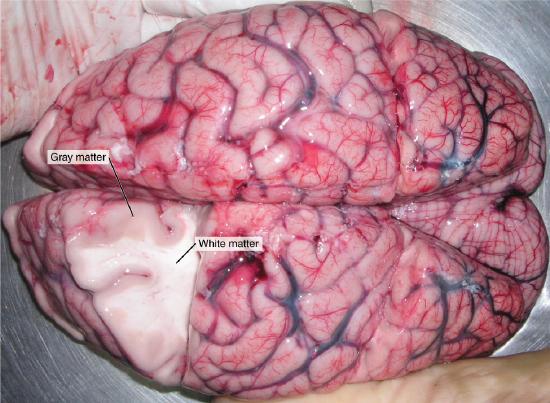
Types of Neurons
There are hundreds of different types of neurons in the human nervous system. These types exhibit a variety of structures and functions. Nonetheless, many neurons can be classified functionally based on the direction in which they carry nerve impulses.
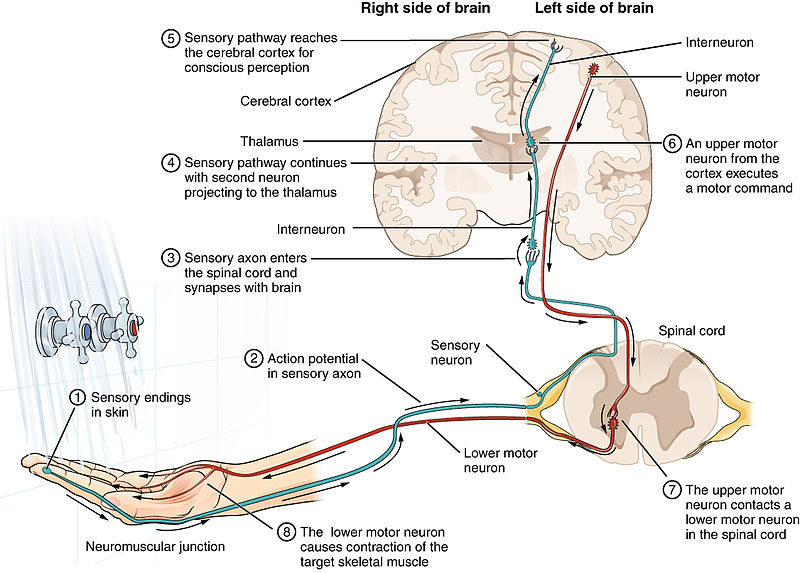
- Sensory (also called afferent) neurons carry nerve impulses from sensory receptors in tissues and organs to the central nervous system. They change physical stimuli such as touch, light, and sound into nerve impulses.
- Motor (also called efferent) neurons, like the one in figure \(\PageIndex{2}\), carry nerve impulses from the central nervous system to muscles and glands. They change nerve signals into the activation of these structures.
- Interneurons carry nerve impulses back and forth often between sensory and motor neurons within the spinal cord or brain.
Glial Cells
Besides neurons, nervous tissues also consist of glial cells (also called neuroglia). They are now known to play many vital roles in the nervous system. There are several different types of glial cells, each with a different function. Schwann cells and Oligodendrocytes are glial cells that produce myelin sheath.
Feature: My Human Body
Would you like your brain to make new neurons that could help you become a better learner? What college student wouldn’t want a little more brainpower when it comes to learning new things? If research on rats applies to humans, then sustained aerobic exercise such as running can increase neurogenesis in the adult brain, and specifically in the hippocampus, a brain structure important for learning temporally and/or spatially complex tasks as well as memory. Although the research is still at the beginning stages, it suggests that exercise may actually lead to a “smarter” brain. However, even if the research results are not confirmed in the future for humans, it can’t hurt to get more aerobic exercise, because it is certainly beneficial for your body if not for your brain.
- Identify the three main parts of a neuron and their functions.
- Describe the myelin sheath and nodes of Ranvier. How does their arrangement allow nerve impulses to travel very rapidly along axons?
- What is a synapse?
- Define neurogenesis. What is the potential for neurogenesis in the human brain?
- Relate neurons to different types of nervous tissues.
- Compare and contrast sensory and motor neurons.
- Identify the role of interneurons.
- A neuron in the spinal cord receives touch information and then transmits that information to another spinal cord neuron that controls the movement of an arm muscle.
- A neuron that takes taste information from your tongue and sends it to your brain.
- A spinal cord neuron stimulates a muscle to contract.
- Sensory neurons
- White neurons
- Peripheral nervous system neurons
- Glial cells
- True or False. Synapses often exist where a dendrite and an axon terminal meet.
- True or False. There is only one axon terminal per neuron.
Explore More
Multiple sclerosis (MS) is a progressive degenerative disease that is caused by the demyelination of axons in the central nervous system. When myelin degrades, the conduction of nerve impulses along the nerve can be impaired or lost, and the nerve eventually withers. Watch this inspirational TED talk in which the speaker shares how being diagnosed with MS changed her life and led her to become an MS nurse.
After his death in 1955, Albert Einstein's brain was studied by scientists worldwide—all wanting to gain insight into the anatomy of a genius. But it wasn't until the 1980s when Marian Diamond noticed that Einstein had more glial cells than average. Glia, stemming from Greek for "glue", was previously thought to have performed a strictly support role for the neurons. Now it is clear that glia may play a more active, non-electrical role in brain activity.
Attributions
- Interneurons of Adult Visual Cortex by Wei-Chung Allen Le e, Hayden Huang, Guoping Feng, Joshua R. Sanes, Emery N. Brown, Peter T. So, Elly Nedivi, licensed CC BY 2.5 via Wikimedia Commons
- Neuron by Chiara Mazzasette adapted from OpenStax , licensed CC BY 4.0 via Wikimedia Commons
- White and gray matter by OpenStax , licensed CC BY 4.0 via Wikimedia Commons
- Sensory Neuron Test Water by OpenStax , licensed CC BY 4.0 via Wikimedia Commons
- Text adapted from Human Biology by CK-12 licensed CC BY-NC 3.0
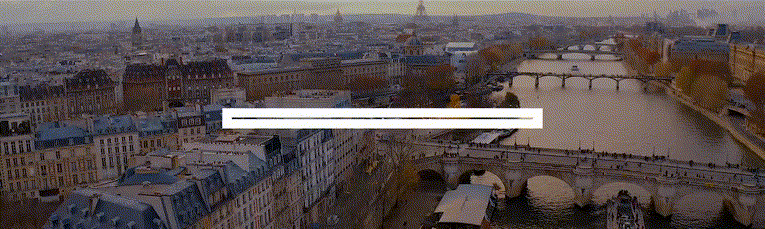
IMAGES
VIDEO
COMMENTS
100. Figure 42.2.2 42.2. 2: The (a) resting membrane potential is a result of different concentrations of Na + and K + ions inside and outside the cell. A nerve impulse causes Na + to enter the cell, resulting in (b) depolarization. At the peak action potential, K + channels open and the cell becomes (c) hyperpolarized.
Figure \(\PageIndex{1}\): Action potential travel along a neuronal axon: The action potential is conducted down the axon as the axon membrane depolarizes, then repolarizes. A node of Ranvier is a natural gap in the myelin sheath along the axon. These unmyelinated spaces are about one micrometer long and contain voltage gated Na + and K + channels.
The axons of peripheral neurons that travel a common route are bundled together to form nerves. Classes of neurons. Based on their roles, the neurons found in the human nervous system can be divided into three classes: sensory neurons, motor neurons, and interneurons. ... If the neuron does end up firing, the nerve impulse, or action potential ...
Neurotransmitters are how we communicate between one cell and the next. Synapses between neurons are either excitatory or inhibitory - and that all comes down to the neurotransmitter released. Excitatory neurotransmitters cause the signal to propagate - more action potentials are triggered. Inhibitory signals work to cancel the signal.
Figure 11.4.4 11.4. 4: An action potential graph of membrane potential over time. A neuron must reach a certain threshold in order to begin the depolarization step of reaching the action potential. The figure also shows the change in potential during the repolarization and refractory periods of the axon.
When an action potential, or nerve impulse, arrives at the axon terminal, it activates voltage-gated calcium channels in the cell membrane. Ca 2 + , which is present at a much higher concentration outside the neuron than inside, rushes into the cell.
Nerve impulses travel just as fast through the network of nerves inside the body. Figure 11.41.1 11.41. 1: The axons of many neurons, like the one shown here, are covered with a fatty layer called myelin sheath. The sheath covers the axon, like the plastic covering on an electrical wire, and allows nerve impulses to travel faster along the axon.
The Brain. Brain anatomy. Each neuron in your brain has one long cable that snakes away from the main part of the cell. This cable, several times thinner than a human hair, is called an axon, and it is where electrical impulses from the neuron travel away to be received by other neurons. Depending on the type of neuron, axons greatly vary in ...
A nerve impulse is a sudden reversal of the electrical charge across the membrane of a resting neuron. The reversal of charge is called an action potential. It begins when the neuron receives a chemical signal from another cell. The signal causes gates in sodium ion channels to open, allowing positive sodium ions to flow back into the cell.
Nervous system - Axon, Signals, Neurotransmitters: The axon arises from the soma at a region called the axon hillock, or initial segment. This is the region where the plasma membrane generates nerve impulses; the axon conducts these impulses away from the soma or dendrites toward other neurons. Large axons acquire an insulating myelin sheath and are known as myelinated, or medullated, fibres.
A nerve impulse is an all-or-nothing response depending on if the stimulus input was strong enough to reach threshold. If a neuron responds at all, it responds completely. A greater stimulation does not produce a stronger impulse. Figure 8.4.2 An action potential speeds along an axon in milliseconds.
An axon is a thin fiber that connects neurons (nerve cells) to that they can communicate. Neurons communicate via electrical impulses that trigger the release of "chemical messengers" called neurotransmitters. Axons also transmit electrical impulses from muscle and gland cells to the brain.
Myelin is made up of lipids and proteins, a fatty substance with a whitish appearance. It is made up of many concentric layers of plasma membrane to make up the myelin sheath around axons. Myelin sheath and myelin function are therefore the same, to increase the speed of nerve impulses.
The neurotransmitter molecules travel across the synaptic cleft and bind to receptors on the membrane of the other cell. If the other cell is a neuron, this starts an action potential in the other cell. ... The nerve impulse travels down the axon membrane as an electrical action potential to the axon terminal.
Saltatory conduction. In neuroscience, nerve conduction velocity (CV) is the speed at which an electrochemical impulse propagates down a neural pathway.Conduction velocities are affected by a wide array of factors, which include age, sex, and various medical conditions. Studies allow for better diagnoses of various neuropathies, especially demyelinating diseases as these conditions result in ...
Anatomy of a neuron. Neurons (or nerve cells) are specialized cells that transmit and receive electrical signals in the body. Neurons are composed of three main parts: dendrites, a cell body, and an axon. Signals are received through the dendrites, travel to the cell body, and continue down the axon until they reach the synapse (the ...
An electrical nerve impulse travels along the axon close axon The long part of a nerve cell along which the electrical signal quickly moves. of the first neuron (presynaptic neuron).; When the ...
Transmission of Nerve Impulses. Neurones transmit electrical impulses, which travel extremely quickly along the neurone cell surface membrane from one end of the neurone to the other. Unlike a normal electric current, these impulses are not a flow of electrons. These impulses, known as action potentials, occur via very brief changes in the ...
Contact: Chen Gu, (614) 292-0349; [email protected]. Written by Emily Caldwell, (614) 292-8310; [email protected]. This image of a neuron illustrates the movement of a nerve impulse along an axon - the long, slender extension of the nerve cell body. Ohio State University researchers have discovered how a key protein finds its way to the right ...
Nerve impulse propagates by jumping from one node of Ranvier to the next. This makes the process of nerve impulse faster as the nerve impulse does not travel the entire length of the axon ( this happens in the case of continuous conduction). The nerve impulse travels at a speed of 100 m/s in saltatory conduction.
Nerve impulses skip from node to node, allowing nerve impulses to travel along the axon very rapidly. A Schwann cell (also on an axon) is a type of glial cell. Its function is to produce the myelin sheath that insulates axons in the peripheral nervous system. In the central nervous system, a different type of glial cell, called an ...
Action Potentials. Neurones transmit electrical impulses, which travel extremely quickly along the neurone cell surface membrane from one end of the neurone to the other. Unlike a normal electric current, these impulses are not a flow of electrons. These impulses, known as action potentials, occur via very brief changes in the distribution of ...
-transport along nerve impulses that travel down the axons -anterograde axonal transport -retrograde axonal transport -travel of the viruses along neurofibrils, Local anesthetics block voltage-gated Na+ channels, but they do not block mechanically gated ion channels. Sensory receptors for touch (and pressure) respond to physical deformation of ...